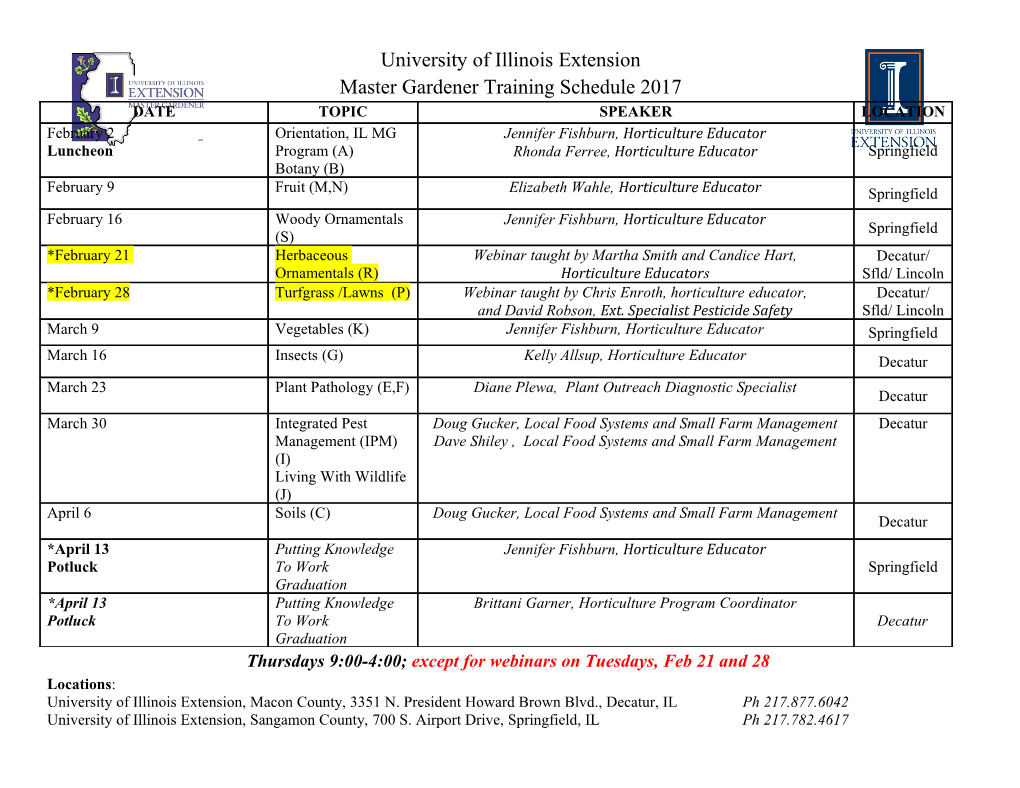
UNITED STATES DEPARTMENT OF THE INTERIOR GEOLOGICAL SURVEY WHERE ON EARTH IS ALL THE LITHIUM? By James D. Vine WITH A SECTION ON URANIUM ISOTOPE STUDIES FISH LAKE VALLEY, NEVADA By J. R. Dooley, Jr. Open-File Report 80-1234 1980 This report is preliminary and has not been reviewed for conformity with U.S. Geological Survey editorial standards. Use of company and trade names is for descriptive purposes and does not constitute endorsement by the U.S. Geological Survey. TABLE OF CONTENTS Introduction 1 A concern for future lithium supplies 1 Evolution of the lithium industry 4 Dispersal of lithium in ancient crystalline rocks 6 Dispersal of lithium in sedimentary rocks, sediments, and waters 10 Defining the problem 12 The search for lithium in unconventional deposits 14 Focus on Cenozoic basins, volcanic activity, and thermal systems in the western United States 14 Drilling for geochemical background data in Clayton Valley, Nevada- 16 Playa deposits of southwestern Nevada 17 Fish Lake Valley 22 Fish Lake Valley uranium isotopic data, by J.R. Dooley, Jr. 24 Columbus Salt Marsh 26 Big Smoky Valley 26 Alkali. Valley 27 Mud Lake 27 Stonewal 1 Fl at 28 Concluding remarks 28 Playa deposits of the California Desert 28 Drilling results 29 Buried salt bodies of Arizona 39 Lithium clays in the Lake Mead and McDermitt areas, Nevada 41 Unusual and speculative lithium deposits 47 Risk reduction in lithium exploration 50 Applicable drilling technology for unconsolidated playa sediments 51 Analysis of the lithium resource problem 52 Introduction 52 Types of lithium deposits having potential economic value 53 Overview 53 Seawater 53 Pegmatites 55 Brines 56 Clays 58 A preliminary classification of lithium anomalies and deposits 63 Lithium anomalies and deposits in the United States 65 Lithium reserves and resources 85 Footnotes to tables 12A, 12B, 12C, 120 91 Looking ahead to a bright future for the lithium industry 93 References cited 96 ILLUSTRATIONS Figure 1. Index map of a part of Esmeralda and Nye Counties, Nevada, showing location of drill sites 18a 2. Index map showing location of bore holes in the California Desert planning area 32 3. Interpretation of data from a lithium exploration program 50a 4. Classification guide for lithium deposits and anomalies 64a 5. Map showing lithium deposits and occurrences of lithium in the United States 65a 11 TABLES Table 1. Conventional uses for lithium 3 2. Physical and chemical properties of lithium 8 3. Lithium-bearing minerals 9a 4. Drilling sites, total depths, and maximum lithium content of samples from wells drilled 19 5. Uranium isotopic water sample analyses 25 6. Lithium content of bore-hole samples from the California Desert playa-evaluation project 30 7. Lithium analyses of bore hole water samples from the California Desert playa-evaluation project 34 8. Physical and chemical properties of some lithium clays 61 9. Lithium-bearing pegmatites, veins, and greisen in the United States 66 10. Lithium-bearing brines and waters 71 11. Lithium deposits and occurrences of rocks of sedimentary and volcanogenic origin in the United States 77 12A. Lithium reserve base and probable yield 87 12B. Lithium inferred reserve base and probable yield 88 12C. Subeconomic and undiscovered in-place resources of lithium and probable yield 89 12D. Summary of geologic in-place reserves and resources of lithium and probable yield 90 Where on earth is all the lithium? James D. Vine, U.S. Geological Survey1 Denver, Colorado 80225 INTRODUCTION A concern for future lithium supplies Hubbert's (1956) forecast of a decline in United States petroleum production coupled with a shortage of gasoline in 1973 provided an important impetus to the search for alternate energy supplies to replace or conserve our dwindling sources of petroleum. Because of their high energy and their power potential per unit weight, lithium batteries are one choice to replace the internal combustion engine for vehicles whose principal use involves a limited daily range. Researchers at Argonne National Laboratory emphasized the significant savings in petroleum if as many as 18 million vehicles were operated with a lithium-sulfur battery by the year 2,000, (Chilenskas, Bernstein, and Ivins, 1976). An additional benefit to be realized by using electric vehicles in place of gasoline powered vehicles includes the reduction in emissions that could be achieved by producing electric power in a central power plant instead of burning gasoline in numerous automobiles. Because of its large power-storage capacity, the lithium battery is also a principal contender for the storage of off-peak power by power companies. Both of these applications (vehicles and power storage) could place large demands on a relatively small lithium industry. Hence, it was logical to question whether or not there will be enough lithium to supply such a vastly increased demand p . Moreover, if the use of lithium in batteries tends to exhaust our resources, the result could have a disastrous effect on the development of thermonuclear (fusion) power for the generation of electricity. The raw material for fusion power consists of deuterium, which occurs naturally in sea water, and tritium, which is produced from lithium by the following reaction: °Li + *n -» ^T + ^He. The Lithium Resource Appraisal Group of the U.S. Geological Survey was organized in 1974 in response to these questions " Retired, present address, 21736 Panorama Drive, Golden, Colorado 80401. ~n dMore recent estimates of the demand have been reduced, in part because other battery couples are expected to be on the market first. Evolution of the lithium industry The lithium industry has undergone a series of developmental changes marked by gradual shifts toward less labor-intensive technology. Prior to 1950 most lithium ore consisted of the hand sorted lithium minerals from zoned pegmatites, such as those in the Black Hills of South Dakota, (Page and others, 1953). Increased demand for lithium initiated by new uses during the war years (1941-45) and by a U.S. Atomic Energy Commission purchase program shortly thereafter, were met by the development of beneficiating techniques that made it possible to concentrate spodumene from unzoned pegmatites in which the average crystal size was less than 10 cm across, (Browning, 1958; McVay and Browning, 1962). Beginning in 1951, the Foote Mineral Company developed an unzoned pegmatite ore body near Kings Mountain, North Carolina, that consisted of a cluster of spodumene-bearing pegmatites--each greater than 15 m thick that could be mined from a single open pit. In the mid-1960's a second ore body of the same type was developed by the Lithium Corporation of American, near Bessemer City, North Carolina, about 6 miles northeast of the Kings Mountain deposit. Meanwhile, a small amount of lithium was produced as a minor byproduct of the extraction of other chemicals from the Searles Lake, California, brine field. This lithium-recovery operation was terminated by Kerr McGee Chemical Company in 1978 after 40 years of production. In the early 1960's, with an increase in demand for lithium, Foote Mineral Company was faced with a need to construct a new facility for extraction of lithium from spodumene or to develop a lithium brine field that had been discovered by the Leprechaun Mining Company at Clayton Valley, near Silverpeak, Nevada. They chose to develop the brine field and produced their first harvest of lithium carbonate in 1967. The simple technique of using a series of solar evaporating ponds to precipitate sodium chloride and to concentrate the lithium into a residual liquor from which lithium can be precipitated as the carbonate, provided an economic advantage over extraction of lithium from spodumene. The increase in demand for lithium during the 1970's caused another shift in company policy, when in 1978 the Foote Mineral Company placed new facilities into operation at Kings Mountain to extract lithium from spodumene. Among the factors considered, no doubt, were: (1) the brine operation was subject to climatic variations that could adversely influence the yield from one year to the next, and (2) several years' experience with the brine field were required before it became apparent from the hydrologic characteristics of the basin that the reserves were less, and the life of the deposit shorter, than was initially anticipated. Hence, any attempt to expand the capacity of the brine operation would have been unwise. The Foote Mineral Company also negotiated with the government of Chile to evaluate the commercial potential of a large lithium- and potassium-rich brine field at Salar de Atacama in the desert area of northern Chile (Comer, 1978). This deposit could thus provide a major source for an expansion of lithium supplies. Among the other potential' major lithium suppliers in the western world, two are of special importance because they could have an effect on the market in the short term. The large zoned pegmatite at Bikita in Zimbabwe (Rhodesia) was a major source for the mineral petalite prior to the 1965 United Nations sanctions against Rhodesia. The sanctions were removed in early 1980 making possible immediate shipment from the Rhodesian stockpile. Thus, the ore from this source could have an immediate impact on the industry. Similarly, ore from several mines in Canada could reach the market in a relatively short time. By the close of the decade of the 1970's, the domestic lithium industry included two major companies, the Lithium Corporation of America, a wholly owned subsidiary of Gulf Resources Corporation of Houston, Texas, and the Foote Mineral Company, more than 90-percent owned by Newmont Mining Company of Danbury, Conn. Both are integrated companies, producing a full line of chemicals from their own ores. Major production of lithium ores outside of the United States includes the Bikita mine in Zimbabwe (Rhodesia), the Karibib-Omaruru area in South-West Africa, U.S.S.R., and China (Singleton, 1979).
Details
-
File Typepdf
-
Upload Time-
-
Content LanguagesEnglish
-
Upload UserAnonymous/Not logged-in
-
File Pages115 Page
-
File Size-