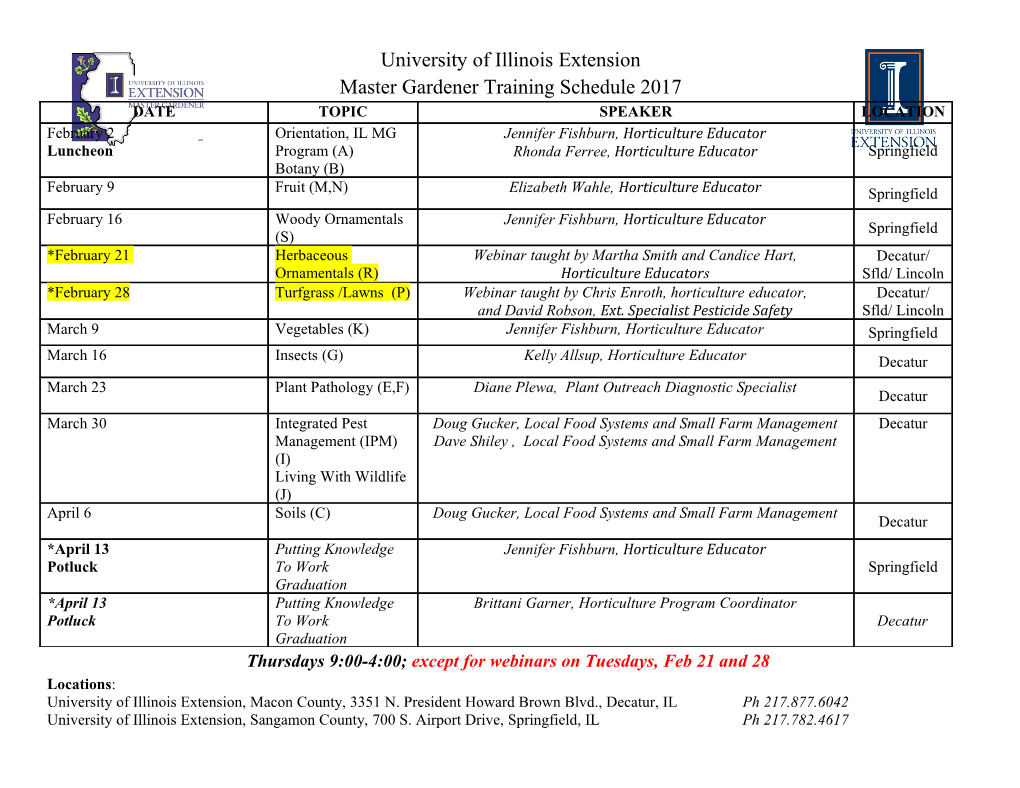
Real Analysis Homework: #1 Yingwei Wang ∗ Department of Mathematics, Purdue University, West Lafayette, IN, USA 1 Banach space ∞ ∞ Question: Let (xn) ⊂ X be a Banach space, and Pn=1 kxnk is convergent. Proof that Pn=1 xn is convergent in X. ∞ ∞ Proof: Suppose Pn=1 kxnk = M < ∞, then ∀ ε> 0, ∃N, s.t. Pn=N kxnk < ε. n Let sn = Pi=1 xi, then ∀n>m>N, n ∞ ∞ ksn − smk = k X xik 6 X kxik < X kxik < ε. i=m i=m i=N Hence, (sn) is a Cauchy sequence and must converge to an element in X. 2 Continuous function Question: f : (X,τX ) → (Y,τY ) is continuous ⇔∀x0 ∈ X and any neighborhood V of f(x0), there is a neighborhood U of x0 such that f(U) ⊂ V . Proof: “⇒”: Let x0 ∈ X f(x0) ∈ Y . For each open set V containing f(x0), since f is −1 continuous, f (V ) which containing x0 is open. Then, there is a neighborhood U of x0 such that −1 x0 ∈ U ⊂ f (V ), that is to say f(U) ⊂ V . “⇐”: Let V ∈ Y . ∀y ∈ V , choose Vy satisfy y ∈ Vy ⊂ V, Vy ∈ τY . Then V = S Vy. −1 Let x = f (y), then ∀Vy, ∃Ux ∈ τX , s.t. x ∈ Ux and f(Ux) ⊂ Vy. Let U = S Ux, then U ∈ τX and f(U)= V . Therefore, f : (X,τX ) → (Y,τY ) is continuous. ∗E-mail address: [email protected]; Tel: 765 337 3504 I Yingwei Wang Real Analysis 3 Closure 3.1 ¯ Question: Topological space (X,τ), x ∈ X, E ⊂ X, x ∈ E ⇔ ∀ neighborhoodV of x, E T V 6= ∅. Proof: Actually, this question is equal to the following one: ¯ (*) Topological space (X,τ), x∈ / E ⇔ There exist an open set V containing x that E T V = ∅. We just need to prove (*). ¯ ¯ “⇒ ” If x∈ / E, then V = X\E is an open set containing x and E T V = ∅. “⇐” If there exist an open set V containing x that E T V = ∅, then X\V is a closed set containing E. By the definition of closer E¯, that is the intersection of all closed sets containing E, the set X\V must contain E. Thus, x∈ / E¯. 3.2 ¯ ∞ Question: Metric space (X, d), x ∈ X, E ⊂ X, x ∈ E ⇔ there is a sequence (en)n=1 in E such that limn→∞ d(en,x) = 0. Proof: Similarly as Section 3.1, the above question is equal to the following one: (**) Metric space (X, d), x∈ / E¯ ⇔ ∀e ∈ E, ∃ ε0, s.t. d(e, x) > ε0. We just need to prove (**). “⇒ ” Suppose x∈ / E¯. Since X\E¯ is an open set, then ∃B(x, δ) ⊂ X\E¯. That is to say ∀e ∈ E, d(e, x) > δ. ε0 “⇐” If ∀e ∈ E, ∃ ε0, s.t. d(e, x) > ε0, then B(x, 2 ) is an open set that containing x and ε0 ε0 B(x, 2 ) T E = ∅. So X\B(x, 2 ) is a closed set containing E. ε0 By the definition of E¯, E¯ ⊂ X\B(x, 2 ). ε0 ¯ Since x ∈ B(x, 2 ), x∈ / E. 4 Distance between point and set If E is a nonempty subset of a metric space X, define the distance from x ∈ X to E by d(x, E)= inf d(x, z). (4.1) z∈E 4.1 (a) Prove that d(x, E) = 0 ⇔ x ∈ E¯. Proof: If infz∈E d(x, z) = 0, then ∀ε, ∃ zε, s.t. d(x, zε) < ε. II Yingwei Wang Real Analysis 1 ∞ Choosing ε = n , then we can get a sequence (zn)n=1 satisfying limn→∞ d(x, zn) = 0. According to Section 3.2, x ∈ E¯. ¯ ∞ “⇐” If x ∈ E, by the previously Section 3.2, there is a sequence (zn)n=1 in E such that limn→∞ d(zn,x) = 0. Then d(x, E) = infz∈E d(x, z) = limn→∞ d(zn,x) = 0. 4.2 (b) Prove that x 7→ d(x, E) is a uniformly continuous function on X, by showing that |d(x, E) − d(y, E)|≤ d(x,y). for all x ∈ X,y ∈ X. Proof: By the definition of (4.1), we can get: ∀x,y ∈ X, ∀ε> 0, ∃zx ∈ E, s.t. d(x, E)= d(x, zx)+ ε, ∃zy ∈ E, s.t. d(y, E)= d(y, zy)+ ε. Without loss of generality, we suppose that d(x, E) ≤ d(y, E). Then when ε is sufficient small, d(y, zy) ≤ d(y, zx). According to triangle inequality, d(x,y) ≥ d(y, zx) − d(x, zx) ≥ d(y, zy) − d(x, zx)= d(x, E) − d(y, E). Besides, in the case that d(x, E) ≥ d(y, E), we can get d(x,y) ≥ d(y, E) − d(x, E). So we can conclude that |d(x, E) − d(y, E)|≤ d(x,y). ε ∀x0 ∈ X, ∀ε > 0, just choose δ = 2 , then ∀x ∈ B(x0, δ), we have |d(x, E) − d(x0, E)| ≤ d(x,x0) <δ<ε. That is to say x 7→ d(x, E) is a uniformly continuous function on X. III Real Analysis Homework: #2 Yingwei Wang ∗ Department of Mathematics, Purdue University, West Lafayette, IN, USA 1 Banach space Question: Let C([a, b]) denote the linear space of continuous function f : [a, b] → R. Show that C[a, b] is a Banach space with respect to the norm kfk = max{|f(t)| : t ∈ [a, b]}. ∞ Proof: Let (fn)n=1 be a Cauchy sequence in C([a, b]). For ∀t ∈ [a, b], ∀m,n ∈ N, |fm(t)−fn(t)|≤ ∞ kfn − fmk, which means (fn(t))n=1 is a Cauchy sequence in R and must converge to an element in R. So we can define a function f : [a, b] → R that f(t) = lim fn(t), ∀t ∈ [a, b]. n→∞ First, we will show that fn → f when n →∞. ∀ε> 0, there is an N s.t. ∀n,m ≥ N we have kfn − fmk < ε. For each x ∈ [a, b] we have |fn(x) − f(x)| = lim |fn(x) − fm(x)| m→∞ ≤ lim kfn − fmk m→∞ ≤ ε, for all n ≥ N. Then for all n ≥ N, kfn − fk = max {|fn(x) − f(x)|} ≤ ε. x∈[a,b] Thus fn → f. ∗E-mail address: [email protected]; Tel: 765 237 7149 I Yingwei Wang Real Analysis Second, we will show that f ∈ C([a, b]). Choose a point t0 ∈ [a, b]. Since fn ∈ C([a, b]), for the previous ε, ∃ δn, s.t. ∀t ∈ (t0 − δ, t0 + δ), |fn(t) − fn(t0)| < ε. For t ∈ (t0 − δ, t0 + δ), n>N we have |f(t) − f(t0)| ≤ |f(t) − fn(t)| + |fn(t) − fn(t0)| + |fn(t0) − f(t0)| < ε + ε + ε < 3ε. Thus f ∈ C([a, b]) and C([a, b]) is a Banach space. 2 Banach space Question: Let l1(N) be the linear space of all the functions f : N → R with the property that ∞ 1 |f|1 := n=1 |f(n)| < ∞. Prove that (l (N), k · k1) is a Banach space. P ∞ 1 Proof: Let (fi)i=1 be a Cauchy sequence in l (N). For ∀n ∈ N, ∀i, j ∈ N, |fi(t) − fj(t)| ≤ ∞ kfi − fjk, which means (fi)i=1(n) is a Cauchy sequence in R and must converge to an element in R. So we can define a function f : N → R that f(n) = lim fi(n), ∀n ∈ N. i→∞ First, we will show that fi → f when i → ∞. ∀ε > 0, there is an I s.t. ∀i, j ≥ I we have kfi − fjk < ε. For each n ∈ N we have |fi(n) − f(n)| = lim |fi(x) − fj(x)| j→∞ ≤ lim kfi − fjk j→∞ ≤ ε, for all i ≥ I. Then for all i ≥ N, kfi − fk = max{|fi(n) − f(n)|} ≤ ε. n∈N Thus fi → f. Second, we will show that f ∈ l1(N). ∞ kfk = |f(n)| 1 X n=1 ∞ = | lim fi(n)| X i→∞ n=1 ∞ ≤ lim |fi(n)| < ∞. i→∞ X n=1 II Yingwei Wang Real Analysis 1 1 Then f ∈ l (N) and (l (N), k · k1) is a Banach space. 3 Application of Baire Theorem Question: Let f : R → R be a smooth function (i.e. C∞). Suppose that for each t ∈ R there is (nt) nt ∈ N such that f (t) = 0. Prove that there is an interval I of positive length such that the restriction of f to I is a polynomial. (n) Proof: Define Tn := {t ∈ R : f (t) = 0}. It is easy to verify that for each n the restriction of f to Tn is a polynomial with at most (n + 1) degree. Then we just need to show that ∃n0, s.t. ◦ (Tn0 ) 6= ∅. c c (n) First, we claim that ∀n ≥ 1, Tn is a closed set. Consider the set Tn = R\Tn: ∀t ∈ Tn, f 6= 0. Since f ∈ C∞, f (n) is continuous, we know that ∃ δ, s.t. ∀x ∈ (t − δ, t + δ), f (n)(x) 6= 0. That is c to say (t − δ, t + δ) ⊂ Tn. Then R\Tn is an open set while Tn is a closed set. ∞ (nt) Second, we claim that Tn = R. ∀t ∈ R, ∃ nt ∈ N, s.t. f (t) = 0, which means t ∈ Tnt . nS=1 ∞ Thus, Tn = R. nS=1 ◦ According to the Baire Category Theorem, ∃n0, s.t. (Tn0 ) 6= ∅. That is to say ∃ interval I ⊂ ◦ (Tn) , s.t. the restriction of f to I is a polynomial. 4 Outer measure Question: Let m∗(A) denote the outer measure of A ⊂ R.
Details
-
File Typepdf
-
Upload Time-
-
Content LanguagesEnglish
-
Upload UserAnonymous/Not logged-in
-
File Pages41 Page
-
File Size-