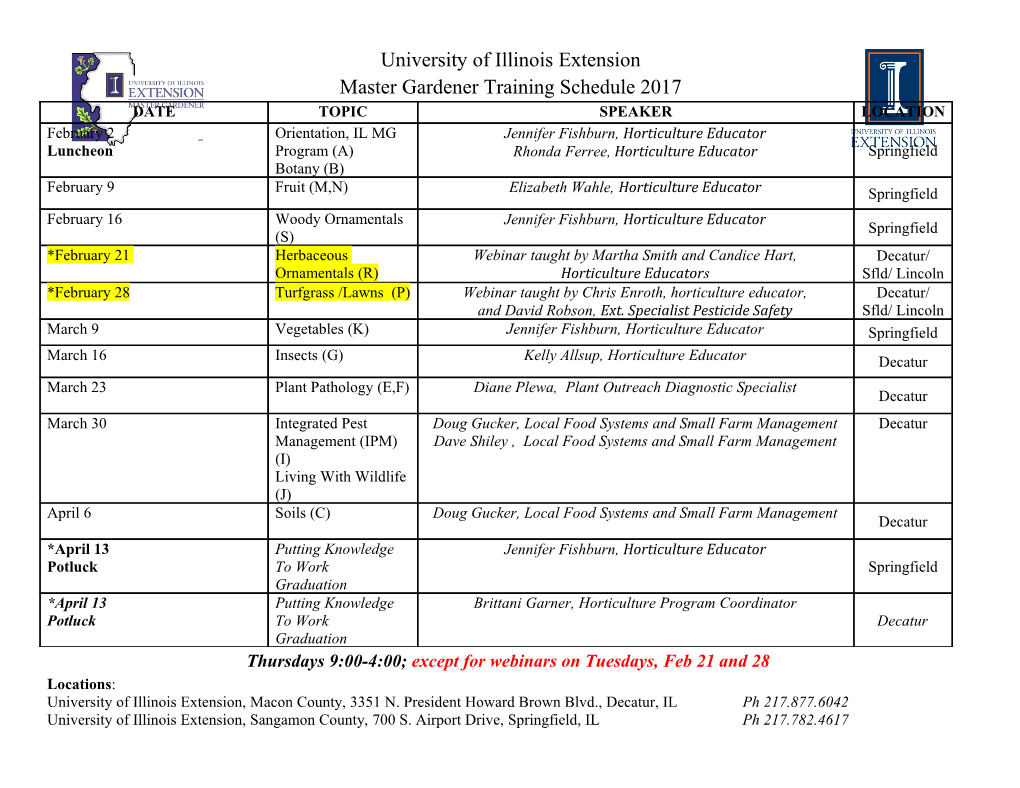
Systemic Delivery of miRNA as a Therapeutic Modality to Treat Cancer John P. Pozniak Submitted in partial fulfillment of the requirements for the degree of Master of Science in Cancer and Cell Biology Committee Chair: Daniel Starczynowski Committee Member: Chunying Du Committee Member: Kathryn Wikenheiser-Brokamp University of Cincinnati Cincinnati, OH April, 2013 Abstract MicroRNAs (miRNA) are small noncoding RNAs with an important role in the initiation and progression of human cancers by posttranscriptional regulation of tumors suppressors and oncogenes. Recent evidence showing miRNAs are aberrantly expressed in tumors underscores them as potential targets for therapeutic intervention. The rationale for developing miRNA-based cancer therapeutics is based on correcting miRNA expression by either antagonism or miRNA replacement in tumors that may provide therapeutic benefit. This article surveys the present knowledge of cancer-associated miRNAs and considers advantages and potential challenges of current delivery technologies employed to systemically deliver miRNAs to tumors by experimental and pre-clinical studies that have used these approaches. Table of Contents 1. Introduction 2. miRNA and Cancer Development 3. Circulating miRNAs as Biomarkers 4. miRNA Therapeutics 5. Efficacy of Systemic Delivery of miRNA With Available Delivery Systems 5.1 Adeno-Associated Virus 5.2 AntagomiRs 5.3 Atellocollagen 5.3 Lipid-Based 5.4 Exosomes 5.5 Non-lipid Nanoparticles 6. Concluding Remarks 7. References Table 1: Summary of Cancer-Associated miRNAs Used Therapeutically in Pre-clinical Models miRNA Chromosome Cancer References Gene Targets References (Expression) miR-15a/16 13q14.2 Prostate (↓) 31 FGF-2, FGFR1, 31 Leukemia (↓) 32 BCL2, CCND1, WNT3A, 34 Lung (↓) 33 CCNE1, CCND3, CDK6 35 miR-34a 1p36.22 Lung (↓) 36 CDK4, CCNE2, MET 47 Prostate (↓) 37 CCND1, CDK6, 48 Breast (↓) 38 BCL2, 36 Pancreas (↓) 39 SIRT1, 49 Colon (↓) 40 YY1, 50 Kidney (↓) 38 BIRC5, 51 Liver (↓) 41 JAG1, WNT1, 52 Bladder (↓) 38 NOTCH1, 53 Skin (↓) 38 LEF1, WNT3, CTNNB1, LRP6, 54 Esophagus (↓) 42 MTA2, TPD52, AXL, 55 Brain (↓) 43 MYCN, 56 Cervix (↓) 44 Ovary (↓) 45 Lymphoid (↓) 46 let-7a 9q22.3 Lymphoid (↓) 57 CCR7 58 Breast (↓) 58 CASP3 59 MYC, 57 DICER1 60 Let-7b 9q22.3 Leukemia (↓) 61 CDK4, CCND1, CCND3, CCNA 62 Skin (↓) 62 miR-26a 3p21.3 Liver (↓) 63 CCND2, CCNE2 65 Lung (↓) 64 PTEN 64 miR-10b 2q31.1 Breast (↑) 66 HOXD10 66 Pancreas (↑) 67 PTEN 68 Brain (↑) 69 miR-143 5q33.1 Colon (↓) 70 HK2, 72 Bone (↓) 71 KLF5, KRAS, BRAF 70 MMP-13 71 miR-145 5q33.1 Colon (↓) 70 KLF5, KRAS, BRAF 70 Breast (↓) 73 P70S6K1 80 Prostate (↓) 74 c-MYC, MUC1 81 Lung (↓) 75 Liver (↓) 76 Bladder (↓) 77 Lymphoid (↓) 78 Ovary (↓) 79 miR-132 17p13.3 Pancreas (↓) 82 RB1 84 Prostate (↓) 83 AChE 85 BTG2, JARID1A, MeCP2, 86 PAIP2A, Ep300 86 MMP-9 87 P120RasGAP 88 miR-33 22q13.2 Colon (↓) 89 PIM-1 89 Lymphoid (↓) 89 ABCA1 90 Introduction MicroRNAs (miRNAs) are ~22 nucleotide (nt) long endogenously expressed short RNA molecules that post- transcriptionally regulate target mRNA by cleavage or repression of their translation1. This subset of non- coding RNA arise from intergenic or intragenic (both exonic and intronic) genomic regions that are transcribed as long primary transcripts that undergo two processing steps to produce a short ‘mature’ and ‘passanger’ strand2. The ‘mature’ strand uses base-pairing to guide RNA-induced silencing complex (RISC) to the target mRNA with full or partial complementary sequences resulting in accelerated exonucleolytic mRNA decay or site-specific endonucleolytic cleavage of the miRNA-mRNA duplex3. miRNAs produced from the ‘passenger’ strand has recently been reported to be important in mRNA regulation and alters the previously held view that miRNA genes produce one functional molecule4. miRNAs are transcribed by RNA polymerase II and processed by Drosha and DGCR8 in the nucleus to yield pre-miRNA which is subsequently exported to the cytoplasm by exportin 5 and processed to a single-stranded miRNA by Dicer5. Complentarity between the “seed” region of miRNA comprising of 2-8 nt and the 3’ UTR of the target mRNA is both necessary and sufficient for the regulation of mRNA6. At present, over 1000 miRNAs are predicted to exist in humans and have been reported to regulate the expression of up to 30% of total cellular proteins7. Most types of human cancers are associated with dysregulation of miRNAs while over half of these genes are found to be located in cancer-associated genomic regions or fragile sites8. Furthermore, recent studies have unveiled the role of miRNAs as master regulators of cancer-signaling networks governing differentiation, proliferation, apoptosis, neovascularization, and stress response9. Because of the large amount of literature on this topic, the scope of this review will refer to aberrantly expressed miRNAs in cancer that have promising clinical applications as candidates for miRNA-based delivery systems. miRNA and Cancer Development The relationship between miRNAs and human malignancies became evident by three important observations. Firstly, miRNAs were shown to regulate proliferation and apoptosis in C. elegans and the fruit fly Drosophilawere, suggesting their deregulation may be implicated in proliferative diseases such as cancer10,11. Secondly, the location of miRNA genes was frequently discovered in fragile sites commonly amplified or deleted in human cancers12. Thirdly, aberrant expression of miRNA genes compared to normal tissue was found linked to malignant tumors13. Since these important observations, accumulating evidence suggests that deregulated miRNAs are a driving-force for tumorigenesis rather than molecular consequence of malignant transformation. Numerous methods for generating comprehensive profiling of miRNA genes of biological and clinical relevance have been developed using microarray, bead-based flow cytometry, and qRT-PCR analysis14. These technologies identify unique signatures or fingerprints of miRNAs in human malignancies and allow researchers to explore new pathways that regulate cancer evolution and enable design of novel diagnostic, prognostic, and therapeutic tools. The first direct evidence of cancer-associated miRNAs was miR-15a and miR-16 that are frequently deleted in chronic lymphocytic leukemia (CLL) and normally induce apoptosis through regulating BCL215. Due to their downregulated status in tumors and role in suppressing the expression of proto-oncogenes, miR-15a and miR-16 were among the first to be classified as tumor suppressor miRNAs. By contrast, oncogenic miRNA (oncomiRs) generally modulate the expression of tumor suppressor genes and are frequently amplified in tumor cells and contribute to tumor development. Importantly, any particular miRNA or cluster of miRNA can have both functions. That is, these terms only reflect state of activity which can vary according to cell type and gene expression pattern16. Furthermore, individual miRNAs can target several components of a single biological pathway or modulate multiple pathways at once. Conversely, each protein-coding gene can be regulated by several miRNAs. This functional versatility provides an advantage of using miRNAs as therapeutic agents to treat cancer patients compared to other RNA inhibition techniques confined to the one-gene-one-target paradigm. The discovery and characterization of miRNAs has provided extraordinary opportunities for the development of new therapeutics to treat cancer. Among the most well studied tumor suppressor miRNAs include miR-34a. Loss of miR-34a expression is observed in a wide range of solid and hematological malignancies contributed by genetic and epigenetic mechanisms (Table 1). Targets of miR-34a largely include components of apoptotic pathways, most notably SIRT1 and BCL217. Several reports demonstrate that restoring expression of miR-34a in mouse tumor xenografts induces tumor regression concomitant with apoptosis and sensitization to chemotherapy and radiation18. These data bolster the concept that miRNAs are promising therapeutic agents and are a requirement for successful cancer therapy. The transition from bench to bedside of a miRNA-based cancer therapy rely on the development of a clinically relevant delivery system that demonstrates robustness, scalable manufacture, and quality controls necessary to support clinical trials. Circulating miRNAs as Biomarkers Accumulating evidence reveals that genetic exchange of miRNA between cells is mediated by encapsulation and transfer through exosomes19. Exosomes are small (50-90 nm) membrane vesicles of endocytic origin that are released into the extracellular milieu by normal and damaged cells20. Exosome secretion is mediated upon exocytic fusion of multivesicular bodies (MVB) with the plasma membrane, a process analogous to the secretion of secretory granules21. Several mechanisms have been hypothesized describing how exosomes interact with their target cells. Exosomes may directly fuse to cells through receptor-ligand interactions and deliver exosomal cargo into the cytoplasm of the recipient cell22. Alternatively, exosomes may be internalized by neighboring cells by endocytic or phagocytic mechanisms23,24. miRNAs have been detected in exosomes released from dendritic cells (DC) which are fused to target DCs following release of exosome content, suggesting a mechanism for DC to DC bidirectional communication and proof-of-concept of exosome-mediated shuttling of miRNA25. Moreover, Mittelbrunn, et. al26, provides evidence that exosomes mediate antigen-driven
Details
-
File Typepdf
-
Upload Time-
-
Content LanguagesEnglish
-
Upload UserAnonymous/Not logged-in
-
File Pages18 Page
-
File Size-