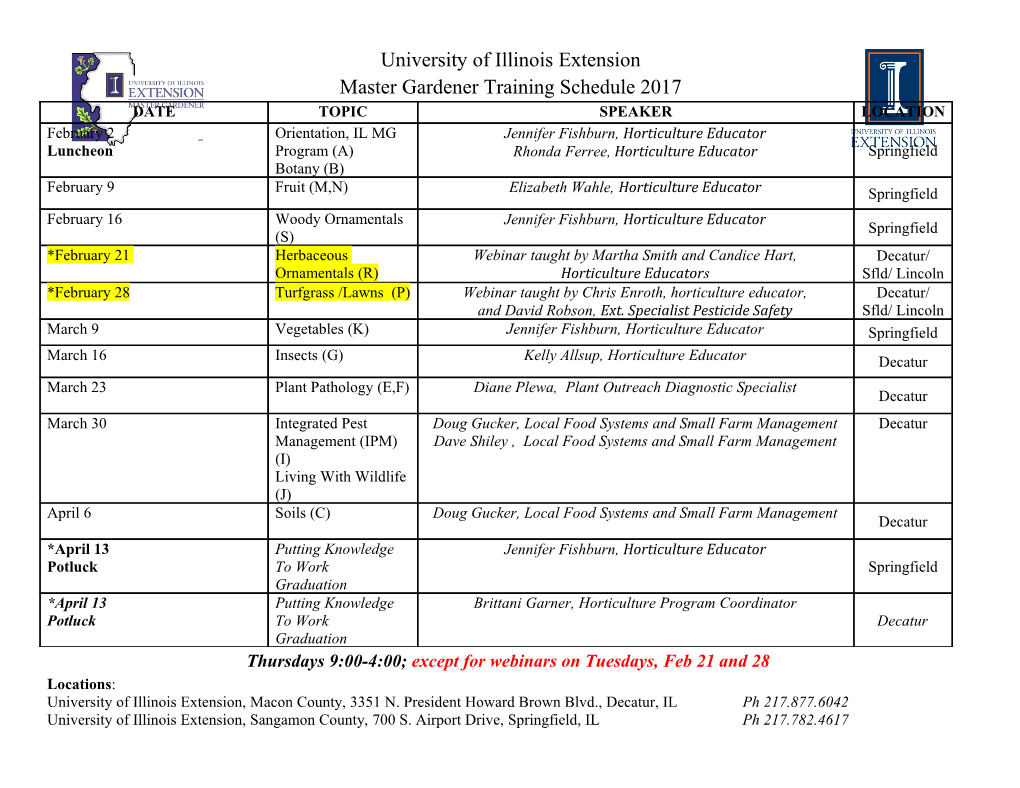
View metadata, citation and similar papers at core.ac.uk brought to you by CORE www.nature.com/scientificreportsprovided by Apollo OPEN Structure of Mycobacterium thermoresistibile GlgE defines novel conformational states Received: 27 July 2015 Accepted: 07 October 2015 that contribute to the catalytic Published: 30 November 2015 mechanism Vitor Mendes1,2, Michal Blaszczyk1, Ana Maranha2, Nuno Empadinhas2 & Tom L. Blundell1 GlgE, an enzyme of the pathway that converts trehalose to α-glucans, is essential for Mycobacterium tuberculosis. Inhibition of GlgE, which transfers maltose from a maltose-1-phosphate donor to α-glucan/maltooligosaccharide chain acceptor, leads to a toxic accumulation of maltose-1- phosphate that culminates in cellular death. Here we describe the first high-resolution mycobacterial GlgE structure from Mycobacterium thermoresistibile at 1.96 Å. We show that the structure resembles that of M. tuberculosis and Streptomyces coelicolor GlgEs, reported before, with each protomer in the homodimer comprising five domains. However, in M. thermoresistibile GlgE we observe several conformational states of the S domain and provide evidence that its high flexibility is important for enzyme activity. The structures here reported shed further light on the interactions between the N-terminal domains and the catalytic domains of opposing chains and how they contribute to the catalytic reaction. Importantly this work identifies a useful surrogate system to aid the development of GlgE inhibitors against opportunistic and pathogenic mycobacteria. Mycobacteria comprise a diverse group of organisms, many of which are known human pathogens. The most notorious member of this genus is undoubtedly Mycobacterium tuberculosis, the major causative agent of tuberculosis (TB), a disease that remains a leading cause of mortality worldwide, claiming an estimated 1.5 million lives in 20131. Furthermore, infections by opportunistic non-tuberculous myco- bacteria (NTM) are increasing worldwide due to their colonization of man-made environments, such as water distribution systems, and their intrinsic detergent and drug resistance that allows them to flourish in hospital environments2. Despite available treatments the rapid emergence of multi-drug and extensively-drug-resistant strains, together with a deadly synergy with HIV, has severely limited our capacity to control and eradicate TB. The combination of the recent increase of NTM infections with the appearance of totally drug-resistant TB strains3 makes the finding of new tools to develop new drugs against these organisms a priority. GlgE is a maltosyl transferase that transfers maltose from a maltose-1-phosphate (maltose-1P) donor to α -(1–4) glucan chains4,5. This enzyme is part of a pathway involving three other enzymes, trehalose synthase (TreS), maltokinase (Pep2/Mak) and glycogen branching enzyme (GlgB), which link trehalose recycling to glycogen and other branched α -glucans synthesis4–6. GlgE is essential for M. tuberculosis and its depletion leads to pleiotropic stress effects and eventually cell death4. Interestingly, cell death is associated with substrate (maltose-1P) hyper-accumulation, and not with the absence of product, 1Department of Biochemistry, University of Cambridge, Cambridge CB2 1GA, UK. 2Molecular Mycobacteriology Group, CNC-Center for Neuroscience and Cell Biology, University of Coimbra, 3004-517 Coimbra, Portugal. Correspondence and requests for materials should be addressed to T.L.B. (email: [email protected]) SCIENTIFIC REPORTS | 5:17144 | DOI: 10.1038/srep17144 1 www.nature.com/scientificreports/ −1 −1 −1 Enzyme Substrate Km (mM) kcat (s ) kcat/Km (M s ) Maltose-1Pa 0.29 ± 0.04 4.06 ± 0.11 14000 ± 2800 M. thermoresistibile Maltoheaxaosea 7.09 ± 0.94 49.91 ± 1.73 7000 ± 1800 Maltose-1Pb 0.25 ± 0.05 1.26 ± 0.07 5000 ± 1000 M. tuberculosis Maltosehexaoseb 35 ± 8 15.4 ± 1.1 440 ± 100 Maltose-1Pc 0.30 ± 0.06 12.3 ± 0.5 41000 ± 8000 S. coelicolor Isoform I Maltohexaosec 1.5 ± 0.3 53 ± 2 36000 ± 7000 Table 1. Comparison of kinetic parameters between M. thermoresistibile, M. tuberculosis and S. Coelicolor GlgEs. aThis study. bObtained from4. cObtained from15. since there are at least two other pathways in M. tuberculosis that lead to glycogen and other α -glucan synthesis4,7. There appears to be complex crosstalk between the GlgE pathway, Rv3032 of the methyl glucose lipopolysaccharide (MGLP) pathway, and glycogen synthase/glucose-1-phosphate adenylyltrans- ferase (GlgA/GlgC) classical glycogen pathway4,8,9, with a synthetic lethal interaction being reported for TreS and Rv30324. This crosstalk seems to be at least partially regulated by phosphorylation, with ser- ine/threonine protein kinase PknB playing a significant role in regulating GlgE activity10. Additionally, the TreS-Pep2-GlgE-GlgB pathway is distributed almost exclusively in bacteria, with rare exceptions in archaea and with no known GlgE orthologues in eukaryotes7. The combined essentiality of GlgE with the lack of orthologues in humans makes it a very attractive target for TB drug discovery. GlgE belongs to the glycosyl hydrolase GH13_3 family11. GH13 enzymes exhibit a conserved 12 three-domain core usually designated by A, B and C . Domain A is a (β /α )8 barrel, while a small domain of variable size (domain B) is inserted between β 3 and α 3 of domain A13. Domain C is a β -sandwich domain located at the C-terminus13. The active site of GH13 enzymes is found in a cleft between domains A and B14. The structure of Streptomyces coelicolor, GlgE isoform I, a 5-domain enzyme forming a dimer, exhibits the typical GH13 core domains with two extra domains at the N-terminus: an α -helix bundle and a second β -sandwich domain15. GlgE is proposed to catalyse the transfer of maltosyl units to α -glucan chains through a double-displacement reaction in which an aspartate residue acts as a nucleophile forming an β -maltosyl-enzyme intermediate, while a glutamate residue is thought to protonate the phosphate and deprotonate the acceptor, acting like an acid/base catalytic residue16. Furthermore, the first GlgE inhib- itors have been reported recently17,18 and the first low resolution M. tuberculosis GlgE structures have just become available19. We here report the first high-resolution X-ray analyses of a mycobacterial GlgE, defining a maltose-GlgE complex of Mycobacterium thermoresistibile GlgE, as well as the apo form and a new conformational state obtained by co-crystallizing GlgE with maltose-1P. We show that M. thermoresistibile GlgE structure resembles that of S. colelicolor and M. tuberculosis GlgEs with each protomer in the dimer comprising five domains, but in M. thermoresistibile GlgE we observe several conformational states of the S domain that point towards considerable flexibility and shed further light on its role in the catalytic mechanism. Results Kinetic properties of M. thermoresistible GlgE. We first determined kinetic parameters in order to compare enzyme properties with M. tuberculosis and with S. coelicolor GlgE, the only orthologous structures available to date. As with M. tuberculosis and S. coelicolor GlgE4,15, M. thermoresistibile GlgE uses maltose-1P exclusively as a maltosyl donor. M. thermoresistible GlgE KM and kcat values are com- parable to those of orthologues from M. tuberculosis and S. coelicolor GlgE (Table 1), with KM values of 0.29 ± 0.04 mM for maltose-1P in the presence of 1 mM maltohexaose and of 7.09 ± 0.94 mM for maltoheaxose in the presence of 5 mM maltose-1P (Supplementary Fig. S1). Both calculated constants are in line with previous observations in M. smegmatis when glycogen was used as a maltosyl acceptor5. M. thermoresistibile GlgE overall structure. The three different structures obtained in this study with resolution limits ranging from ~2.0 to ~3.3 Å were GlgE apo-form (5CJ5), GlgE-maltose com- plex (5CGM) and a second GlgE-maltose complex (5CIM) obtained by co-crystallizing with GlgE with maltose-1P. Of the three different tags (C-terminal non-cleavable 6xHis Tag, N-terminal non-cleavable 6xHis Tag and N-terminal cleavable SUMO tag) used, the construct with an N-terminal cleavable SUMO tag produced the best diffracting crystals and was used to obtain crystals in all conditions. However, we could only obtain high-resolution crystals (~2.0 Å) when GlgE was co-crystalized with maltose, maltohexaose or both. Apo-form crystals and GlgE-maltose-1P co-crystallizations always produced crystals that diffracted to lower resolutions (< 3 Å) despite all optimization efforts. The maltohexaose and maltose co-crystallizations produced indistinguishable structures in which only maltose could be observed at the active site. This was not unexpected since others have reported that GlgE slowly degrades SCIENTIFIC REPORTS | 5:17144 | DOI: 10.1038/srep17144 2 www.nature.com/scientificreports/ Dataset Maltose Maltose-1-P APO Data collectiona Beamline at DLS I04–1 I04 I24 Wavelength (Å) 0.92 0.979 0.978 Space group P212121 P212121 P6 a = 80.33; a = 77.5; b = 112.9; a = b = 197.64; Unit cell dimensions (Å) b = 113.90; c = 221.4 c = 105.62 c = 220.50 54.3–1.92 221.4–3.32 171.2–3.13 Resolution range (Å) (2.02–1.92) (3.50–3.32) (3.30–3.13) Reflections (measured/unique) 147,668/10,554 149,129/28,988 563,574/41.587 Completeness (%) 99.9 (99.9) 99.8 (99.8) 99.7 (99.7) Multiplicity 12.8 (13.7) 10.9 (8.6) 13.5 (13.6) b Rsym 0.065 (0.690) 0.083 (0.595) 0.218 (1.541) Mean [(I)/σ (I)] 11.4 (2.44) 5.0 (5.2) 13.0 (2.3) Protomers per asymmetric unit 2 2 2 Matthews coefficient (Å3 Da−1) 3.25 3.26 3.47 Solvent content (%) 62.1 62.5 67.4 Refinement Resolution range (Å) 37.74–1.95 100.6–3.32 57.06–3.13 c d Rfactor /Free Rfactor (%) 17.5/20.2 17.8/21.4 25.9/30.8 Unique reflections (working/ 10,227/564 29,449/1494 41621/2093 test set) Water molecules 934 116 0 Total number of atoms 11650 10246 10489 r.m.s.d.
Details
-
File Typepdf
-
Upload Time-
-
Content LanguagesEnglish
-
Upload UserAnonymous/Not logged-in
-
File Pages10 Page
-
File Size-