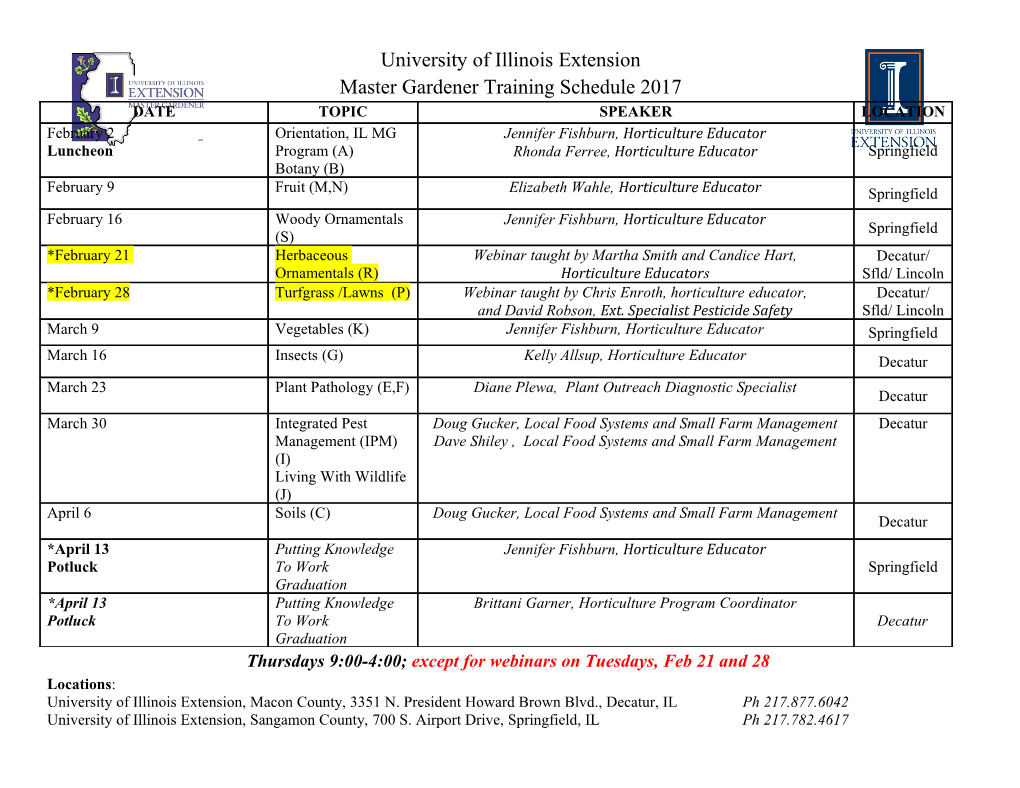
ORE Open Research Exeter TITLE Quinol-cytochrome c oxidoreductase and cytochrome c4 mediate electron transfer during selenate respiration in Thauera selenatis AUTHORS Lowe, EC; Bydder, S; Hartshorne, RS; et al. JOURNAL Journal of Biological Chemistry DEPOSITED IN ORE 08 May 2013 This version available at http://hdl.handle.net/10871/9201 COPYRIGHT AND REUSE Open Research Exeter makes this work available in accordance with publisher policies. A NOTE ON VERSIONS The version presented here may differ from the published version. If citing, you are advised to consult the published version for pagination, volume/issue and date of publication QUINOL-CYTOCHROME C OXIDOREDUCTASE AND CYTOCHROME C4 MEDIATE ELECTRON TRANSFER DURING SELENATE RESPIRATION IN THAUERA SELENATIS* Elisabeth C. Lowe1, Sarah Bydder2, Robert S. Hartshorne3, Hannah L.U. Tape1, Elizabeth J. Dridge1, Charles M. Debieux1, Konrad Paszkiewicz1, Ian Singleton4, Richard J. Lewis5, Joanne M. Santini6, David J. Richardson3 and Clive S. Butler1 1School of Biosciences, Centre for Biocatalysis, University of Exeter, Stocker Road, Exeter EX4 4QD, UK 2Department of Microbiology, La Trobe University, 3086 Victoria, Australia 3School of Biological Sciences, University of East Anglia, Norwich NR4 7TJ, UK 4Institute for Research on Environment and Sustainability, University of Newcastle, Newcastle upon Tyne NE2 4HH, UK 5Institute for Cell and Molecular Biosciences, University of Newcastle, Newcastle upon Tyne NE2 4HH, UK 6Institute of Structural and Molecular Biology, UCL, Gower Street, London WC1E 6BT, UK Running head: Pathways of electron transfer in T. selenatis Address correspondence to: Clive S. Butler, School of Biosciences, Centre for Biocatalysis, University of Exeter, Stocker Road, Exeter, UK. EX4 4QD. Fax: +44 1392 263434; E-mail: [email protected] Selenate reductase (SER) from Thauera selenatis is a periplasmic enzyme that has been Within the DMSOR1 family of type II classified as a type II molybdoenzyme. The molybdoenzymes (1) there is a distinct clade of enzyme comprises three subunits SerABC, enzymes that are translocated to the periplasm where SerC is an unusual b-heme cytochrome. using the twin arginine translocation (TAT) In the present work the spectropotentiometric pathway (2,3) and possess a monomeric b-type characterization of the SerC component and heme-containing γ-subunit (1). The enzymes the identification of redox partners to SER are within this clade function as either reported. The mid-point redox potential of the dehydrogenases (e.g. ethylbenzene dehydrogenase b-heme was determined by optical titration (Em from Aromatoleum aromaticum (4), +234±10 mV). A profile of periplasmic c-type dimethylsulphide dehydrogenase from cytochromes expressed in T. selenatis under Rhodovulum sulfidophilum (1,5) or reductases selenate respiring conditions was undertaken. (e.g. selenate reductase from Thauera selenatis Two c-type cytochromes were purified, (~24 (6,7), chlorate reductase from Ideonella kDa and ~6 kDa), and the 24 kDa protein (cytc- dechloratans (8,9), and catalyze either hydride or Ts4) was shown to donate electrons to SerABC oxygen transfer as generalized by equation [1]. in vitro. Protein sequence of cytc-Ts4 was + - obtained by N-terminal sequencing and LC- X + H2O ' XO + 2H + 2e [1] MS/MS analysis, and based upon sequence similarities, was assigned as a member of These soluble enzymes consist of three cytochrome c4 family. Redox potentiometry, subunits and in addition to the b-heme combined with UV-visible spectroscopy, cytochrome (γ-subunit), they comprise an iron- showed that cytc-Ts4 is a diheme cytochrome sulfur protein (β-subunit) co-ordinating 1x[3Fe- with a redox potential of +282±10 mV and both 4S] cluster and 3x[4Fe-4S] clusters, and a hemes are predicted to have His-Met ligation. catalytic component (α-subunit) that co-ordinates To identify the membrane-bound electron a [4Fe-4S] cluster and the active site molybdenum donors to cytc-Ts4, growth of T. selenatis in the presence of respiratory inhibitors was monitored. The specific quinol-cytochrome c 1 MGD, molybdenum guanine dinucleotide; SER, oxidoreductase (QCR) inhibitors myxothiazol periplasmic selenate reductase; NAR, membrane- and antimycin A partially inhibited selenate bound nitrate reductase; EBDH, ethylbenzene respiration, demonstrating that some electron dehydrogenase; DMSDH, dimethyl sulfide flux is via the QCR. Complete inhibition of dehydrogenase; DMSOR, dimethylsulfoxide reductase; selenate respiration was achieved with the FDH, formate dehydrogenase; FeS/FS0, iron-sulfur more general inhibitor 2-n- heptyl- 4- clusters; HQNO, 2-n-heptyl-4-hydroxyquinoline N- hydroxyquinoline N-oxide (HQNO). Electron oxide; TMAOR, trimethylamine N-oxide reductase; TAT, twin-arginine translocation; QCR, quinol- transfer via a QCR and a diheme cytochrome cytochrome c oxidoreductase; QDH, quinol c4 is a novel route for a member of the DMSO dehydrogenase; DH, primary dehydrogenase; PMF, reductase family of molybdoenzymes. proton-motive force; OFN, oxygen free nitrogen. 1 guanine dinucleotide (MGD) cofactor (10,11) high potential b-heme in γ-subunits of both EBDH (Figure 1). The reductases play a pivotal and DMSDH, that the γ-subunit is the site of function, coupling the reduction of substrates to electron egress from these enzymes to their redox the generation of the proton motive force (PMF). partners (10,15). Consequently, the high potential Identifying the route by which electrons are b-heme might function to draw electrons away transferred to these reductases is vital to from, rather than towards, the MGD cofactor understanding their bioenergetics (12). How during catalysis as displayed in the nitrate periplasmic substrate reduction can generate a reductase enzymes. Analysis of the amino acid PMF, that is sufficient to support growth, is of sequence alignment of EBDH and DMSDH with considerable interest. The use of selenate and selenate reductase (SER) shows that the co- selenite as bacterial respiratory substrates has ordinating methionine (M138) and lysine (K228) been well documented (13). By far the most well residues are also conserved in SerC. This then studied selenate-respiring bacterium has been T. raises interesting questions as to what is the redox selenatis; however, the characterisation of the potential for the b-heme in the reductase members selenate respiratory system has not yet addressed of this distinct type II clade and what are the the mechanism by which electrons are transferred likely redox partners? from the cytoplasmic membrane to the Among the related type II molybdoenzymes, periplasmic selenate reductase (SerABC). As there are a number of different routes of electron SerABC appears to be soluble and not associated transfer to terminal reductases, although the with the inner or outer membrane (6), it is likely majority catalyze reactions with a lower redox that another protein acts as a shuttle to take potential than the selenate/selenite couple (+475 electrons from the membrane and deliver them to mV). For example; the DMSO/DMS couple is the b-heme of SerC. Reduced SerC then donates +160 mV and TMAO/TMA couple is +130 mV electrons, via the iron-sulfur clusters of SerAB (17). Within the DMSOR group, a number of (11), specifically to the molybdopterin co-factor pathways for electron transport have been 2- for reduction of selenate (SeO4 ) to selenite established, and a variety of membrane-bound 2- (SeO3 ) (6,14,11). proteins have been implicated. These membrane- The b-heme of SerC is presumed to be the site bound cytochromes are responsible for extracting of electron entry to the SER enzyme complex, but electrons from the quinol pool so that they can be also remains poorly studied. The recently solved transferred to the associated downstream terminal crystal structure of ethylbenzene dehydrogenase reductases. The existing literature provides (EBDH) reveals that its γ-subunit also binds an several routes by which electrons are transferred. unusual b-type heme, which is coordinated by For example, the membrane bound nitrate methionine and lysine ligands (15). This co- reductase NarGH (16,18) receives its electrons ordination favours the uncharged ferrous heme from its dedicated NarI membrane integral over the charged ferric form and as such subunit, which contains two b-hemes; the DMSO modulates a relatively high redox potential of reductase from E. coli (DmsABC) has a similar +254 mV. Similarly, optical potentiometeric organisation of subunits (17), but faces the redox titrations of the heme b in the γ-subunit of periplasm. The E. coli TMAO reductase TorA dimethylsulphide dehydrogenase (DMSDH) (Rhodobacter sp.), DMSO reductase DorA (17), revealed a high redox potential of Em = +324mV and the periplasmic nitrate reductase NapAB (19) (10). By contrast, the cytoplasmic-facing accept electrons from dedicated tetra or penta- respiratory nitrate reductase NAR from heme quinol dehydrogenases in the membrane Escherichia coli (another member of the type II (TorC/DorC/NapC). In the case of Nap, there is molybdoenzymes) possesses a membrane bound also an alternative pathway; the nap gene cluster γ-subunit (NarI) that co-ordinates two bis-His in E. coli for example, also contains napG and ligated b-hemes that have much lower redox napH, which encode iron-sulfur proteins potentials at +20 and +120 mV (16). In the case responsible for electron transfer from ubiquinol to of NAR, the low redox potentials of the heme NapC (20). favours electron flow away from NarI, towards It may be the
Details
-
File Typepdf
-
Upload Time-
-
Content LanguagesEnglish
-
Upload UserAnonymous/Not logged-in
-
File Pages25 Page
-
File Size-