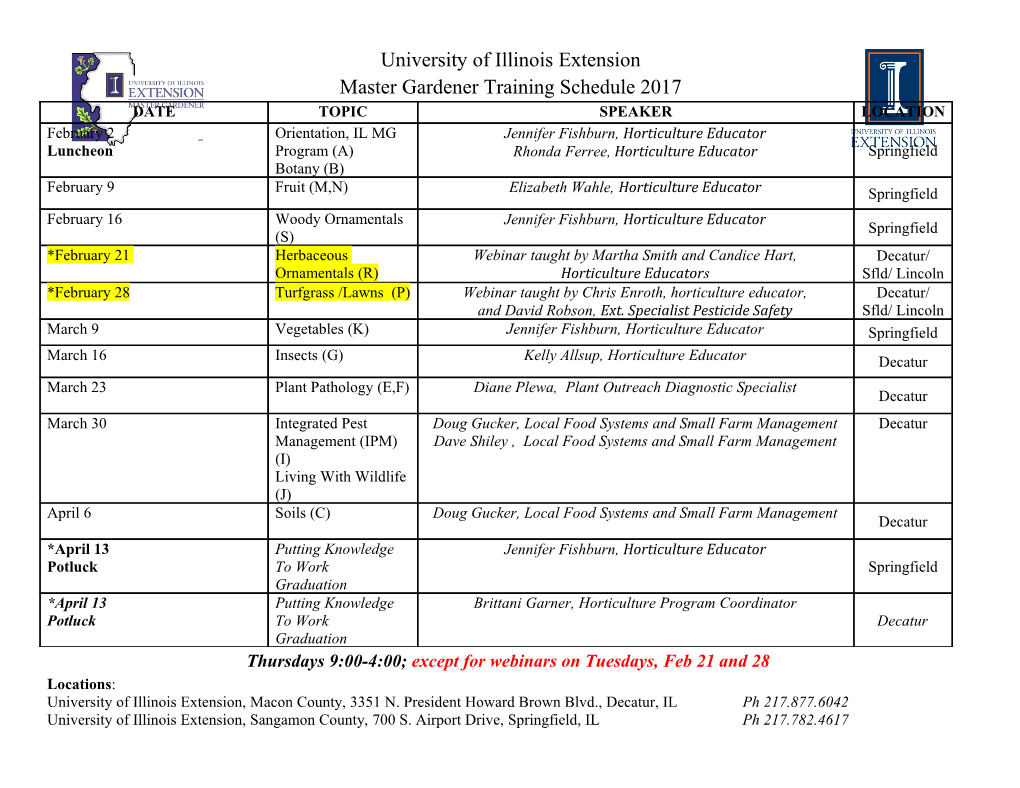
ABSTRACT COMPLEX RELATIONSHIPS AMONG WATERSHED LAND COVER AND RESERVOIR MORPHOMETRY, PRODUCTIVITY, AND ZOOPLANKTON COMMUNITIES by Elisabeth J. Hagenbuch Proper management of aquatic systems requires knowledge of the links among landscape-level anthropogenic disturbances, terrestrial subsidies, and aquatic ecosystem properties. Due to their large watershed area to surface area ratios, reservoirs often receive substantial subsidies of nutrients and sediments. To better understand relationships between environmental variables and reservoir ecosystem properties, we examined relationships among variables representing landscape-level features, reservoir morphometry, water quality parameters and zooplankton community biomass for 109 reservoirs spanning a wide productivity gradient. A principal components analysis (PCA) identified two significant environmental gradients, the first representing reservoir productivity and land use cover and the second representing reservoir morphometry. A regression tree analysis used landscape-level and morphometric parameters to classify reservoirs according to their productivity level into four groups. Significant correlations were detected between small-bodied zooplankton (rotifers and/or copepod nauplii) and several environmental parameters. Non-metric multidimensional scaling plots identified a separation between reservoirs in the highest and lowest productivity groups, but only for rotifer communities. COMPLEX RELATIONSHIPS AMONG WATERSHED LAND COVER AND RESERVOIR MORPHOMETRY, PRODUCTIVITY, AND ZOOPLANKTON ASSEMBLAGES Submitted to the Faculty of Miami University in partial fulfillment of the requirements for the degree of Master of Science Department of Zoology by Elisabeth J. Hagenbuch Miami University Oxford, Ohio 2010 Advisor_______________________________ María J. González Reader________________________________ Martin H. H. Stevens Reader________________________________ Michael J. Vanni Reader________________________________ William H. Renwick TABLE OF CONTENTS List of Tables iii List of Figures iv Acknowledgements v Introduction 1 Methods 4 Results 9 Discussion 12 References 30 Appendices 34 ii LIST OF TABLES Table 1 19 Table 2 20 Table 3 21 Table 4 21 Table 5 22 Table 6 23 iii LIST OF FIGURES Figure 1 24 Figure 2 25 Figure 3 26 Figure 4 27 Figure 5 28 Figure 6 29 iv Acknowledgements Foremost, I would like to express my appreciation to my advisor, María González. María provided consistent direction for this project and without her help and encouragement this project would not have been possible. Each member of my graduate committee—Hank Stevens, Mike Vanni, and Bill Renwick—were integral in that they each provided assistance on a specific area of expertise within this project. In addition, I would like to thank Jon Denlinger and Scott Hale of the Ohio Department of Natural Resources Division of Wildlife for working toward the grant which made this project possible. The initial sample kit organization, preparation, collection, and processing was performed by Lesley Knoll. I would also like to thank Robbyn Abbitt and Stacy Xanakis for their help with GIS and Joe Conroy for his valuable draft comments. Funding for this research was provided by the Ohio Department of Natural Resources Division of Wildlife (Project: FADR48) and Miami University’s Summer Workshop Grant (Department of Zoology). v Introduction In an effort to understand the increasingly global effects of eutrophication on aquatic ecosystems, relationships between nutrients and primary productivity have been heavily studied (Smith 2003, Schindler 2006, Smith and Schindler 2009). However, in order to effectively inform management strategies, it is also crucial to identify links between anthropogenic landscape-level disturbances, such as agriculture (e.g., Knoll et al. 2003) and urbanization (e.g., Dodson et al. 2005, Gélinas and Pinel-Alloul 2008), and indicators of eutrophication. Effects of anthropogenic disturbance may be more pronounced in human-made reservoirs, which tend to have smaller surface water areas (Whittier et al. 2002) and larger watershed area:surface water area ratios (WA:SA) when compared to natural lakes (Kimmel et al. 1990). Consequently, relative to natural lakes, reservoirs receive proportionately greater subsidies of nutrients and sediments (Kimmel et al. 1990, Vanni et al. 2005). Reservoirs are essential for supplying water for crop irrigation, livestock, drinking water, and recreation in many areas, and their numbers are increasing worldwide (Downing et al. 2006). Furthermore, predicted patterns for worldwide human population growth and the associated need for increased food production will result in the conversion of available land for agricultural crop and pasture use and be accompanied by increases in nitrogen and phosphorus fertilizer usage (Tilman et al. 2001). In addition, recent demands for alternative fuel sources, such as grain-based ethanol, may increase rates of land cover conversion and nutrient subsidies to aquatic systems (Simpson et al. 2008). Given the substantial increases in both the number of reservoir impoundments and conversion of land for agricultural uses, it is surprising that few studies have looked specifically at landscape-level influences on reservoir ecosystems (but see Knowlton and Jones 2000, Knoll et al. 2003, Jones et al. 2004, Bremigan et al. 2008, Hale et al. 2008). Recent syntheses show that many reservoirs in the United States suffer from symptoms of eutrophication, including elevated concentrations of nutrients and phytoplankton (Whittier et al. 2002, Dodds et al. 2009). However, the internal and external factors driving trophic status are not well known. As in natural lakes, positive correlations exist between indicators of reservoir eutrophication such as high concentrations of total phosphorus (TP), total nitrogen (TN) and chlorophyll concentrations (Knoll et al. 2003, Jones et al. 2004). In addition, total phosphorus concentration is related to the extent of agricultural land cover and watershed area:water body volume ratios (Knoll et al. 2003). Row-crop agriculture has been linked to higher TN:TP ratios, 1 whereas this ratio was lower in agricultural areas containing primarily pasturelands and animal operations (Arbuckle and Downing 2001). In addition, reservoir trophic status and watershed size have been used to explain variation in fish biomass (Hale et al. 2008). In 11 Ohio reservoirs, models including agricultural land use and reservoir depth explained the most variation in nutrient concentrations and chlorophyll, while zooplankton biomass was only related to chlorophyll (Bremigan et al. 2008). Zooplankton represent a key group in reservoir food chains, linking phytoplankton and fish, especially during fish larval and juvenile stages (Welker et al.1994, Bremigan and Stein 1997, Bunnell et al. 2003, Bremigan et al. 2008). Thus, in reservoirs spanning a large gradient of productivity, information on factors explaining variation in zooplankton communities can provide fisheries managers with information on potential available prey for fish (Stein et al. 1995). In addition, zooplankton communities have potential as biological indicators of lake trophic status (Gannon and Stemberger 1978, Bays and Crisman 1983). Positive relationships have been identified between total crustacean biomass and productivity in natural lakes and reservoirs (Pinto-Coelho et al. 2005, Pace 1986, Gyllström et al. 2005). In oligotrophic lakes, crustacean biomass increases in conjunction with residential cover, total phosphorus and total nitrogen (Gélinas and Pinel-Alloul 2008). Several studies have identified a unimodal relationship between primary productivity and crustacean species richness (Dodson et al. 2000, Mittelbach et al. 2001, Hoffmann and Dodson 2005) and changes in crustacean richness have been associated with indirect effects of riparian landscape-level changes (Dodson et al. 2005). Similarly, rotifer (Whitman et al. 2004, Yoshida et al. 2003) and microzooplankton biomass (including rotifers and nauplii; Bays and Crisman 1983) have been positively related to lake productivity. Changes in rotifer species composition have been documented along a trophic gradient (Duggan et al. 2001, Yoshida et al. 2003). However, studies using both rotifer and crustacean zooplankton illustrate the possible contrasting responses of different zooplankton groups to altered trophic conditions. Stemberger and Lazorchek (1994) observed that nutrient-poor lakes were dominated by large cladocerans and calanoid copepods whereas nutrient-rich lakes contained small cladocerans, cyclopoid copepods, nauplii, and rotifers. Similarly, rotifer and small cladoceran abundance (Whitman et al. 2004) and microzooplankton biomass (including rotifers and nauplii; Bays and Crisman 1983) have been positively related to increasing lake productivity. In temperate Ohio reservoirs, phytoplankton biomass (chlorophyll) was negatively related to 2 crustacean biomass, but positively related to rotifer biomass (Bremigan et al. 2008). Such variable responses demonstrate the benefit of including both rotifers and crustaceans in predictive analyses. In this study, we wished to examine how landscape-level variables and within-reservoir physical features can predict reservoir water quality and zooplankton communities. We first analyzed relationships among three categories of environmental parameters: 1) water quality (chlorophyll, suspended solids and nutrients), 2) morphometric (maximum depth, surface area), and 3) landscape-level
Details
-
File Typepdf
-
Upload Time-
-
Content LanguagesEnglish
-
Upload UserAnonymous/Not logged-in
-
File Pages42 Page
-
File Size-