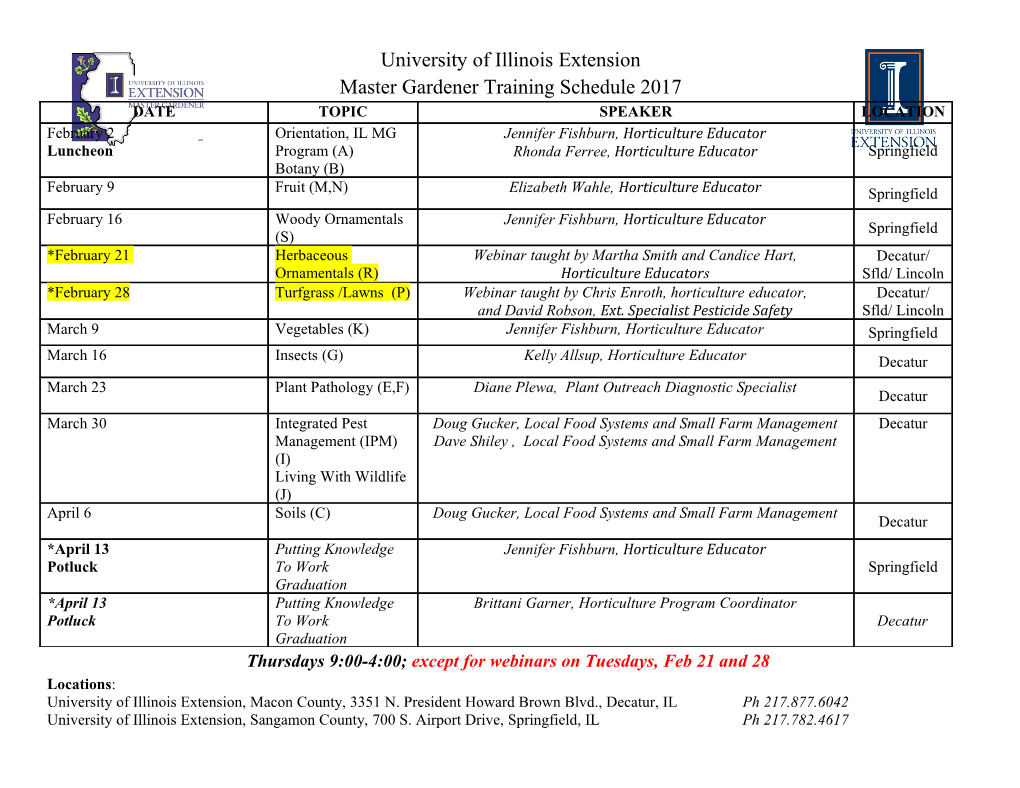
Analysis of the Domain Specific Function of the Wiskott Aldrich Syndrome Protein, in Vitro and in Vivo By Austen Jonathan Jacob Worth A thesis submitted for the degree of Doctor of Philosophy 2009 1 Abstract Wiskott Aldrich Syndrome (WAS) is an X-linked immunodeficiency characterised by low numbers of low volume platelets, eczema, severe immunodeficiency and increased susceptibility to malignant and autoimmune diseases. Wiskott Aldrich Syndrome protein (WASp), the product of the gene mutated in WAS is a multidomain cytosolic protein which acts by integrating downstream signals from cell surface receptor signalling cascades and transduces these signals into remodelling of the actin cytoskeleton. WASp is involved in cellular processes as diverse as cell polarisation, migration, phagocytosis and T cell synapse formation. Dendritic cell function and migration is known to be impaired in WAS. Specifically, dendritic cells lacking WASp in both mice and humans show an impaired ability to form transient actin rich adhesive structures called podosomes. In this thesis, I am presenting the characterisation of a panel of domain deletion WASp proteins and a series of point mutations which, in children give rise to a range of disease phenotypes. I have expressed these constructs as GST tagged proteins and developed a bead based in vitro assay to asses the protein activity. I have compared the ability of these mutants to bind key regulators of WASp function, WIP and Cdc42, using a variety of immunoprecipitation techniques. Finally I have expressed EGFP tagged WASp proteins in myeloid cell lines to assess expression and stability of these constructs and in WASp null murine dendritic cells to assess their ability reconstitute podosomes. Point mutations which cause WAS have slightly increased actin polymerisation activity compared to wild type WASp (WT) and have a normal affinity for Cdc42. Although they have a reduced affinity for WIP compared to WT, there is still significant association of WIP to mutant WASp in cells. These mutants have reduced stability and different patterns of phosphorylation compared to WT, when expressed in myeloid cells lines. The EVH1, Polyproline and VCA domains of WASp are all essential for optimal actin polymerisation activity, whereas the Basic domain and the point mutation H246D (which inhibits Cdc42 binding) could be deleted with no loss of molecular activity. The EVH1 domain is essential for WIP binding and the Basic domain is essential for Cdc42 binding. Deletion of the EVH1 domain resulted in protein which had greater stability than WT WASp, suggesting that residues within the EVH1 domain are essential for the physiological degradation of WASp. This work demonstrates that mutant WASp which causes WAS in patients has normal molecular activity, but the regulation of mutant WASp degradation is impaired in myeloid cells. Results from this project also suggest that Cdc42 is not essential for normal WASp functioning, but does appear to be essential for the phosphorylation of the Y291 residue, which is known to be critical for the regulation of WASp activity. Further work will aim to elucidate the functional (in vivo) significance of these findings. 2 Acknowledgements I would like to thank all the members of the Molecular Immunology Unit at the Institute of Child Health for their help and patience during my three years work in the lab. In particular Gerben Bouma, Mike Blundell, Dale Moulding and Joao Metelo have been a constant source of advice, help, learning and encouragement, and Sue Ballard’s continual practical assistance has been invaluable. I would like to thank my collaborators for their help and useful discussion of my results. This includes Gareth Jones, Yolanda Calle, David Adamovich, Scott Snapper and particularly Giles Cory, who gave me an invaluable grounding at the start of my project. I would also like to acknowledge several colleagues from other laboratories who have kindly provided me with reagents or protocols. These include Ines Anton, Marc Krishner, Narayanaswamy Ramesh, Ritu Garg and Anne Ridley. I must give particular thanks to my supervisors Siobhan Burns and Adrian Thrasher, and to Christine Kinnon, head of the Molecular Immunology Unit, for their scientific, academic and professional help and advice. Siobhan in particular, has been a fantastic support, providing exemplary supervision to a difficult and single minded student. Finally I would like to thank my family, particularly Perys Worth, for their love and support during a difficult time completing this project. My greatest thanks, however, must be reserved for my wife, Louise, and son, Jonathan, without whose unerring love and support none of this would have been possible. Declaration All the work presented in this thesis is entirely my own with the following exceptions; (1) The Y291E and I294T WASp constructs were generated by Giles Cory and were a gift to me. (2) Cell sorting of U937 cells into high and low expressing cells was performed by Joao Metalo. (3) The counting of podosomes for figure 9.1e was performed by Joao Metelo and Siobhan Burns. One of the two DC preparations for this data was performed by myself, the other by Joao Metelo. 3 Publications Blundell,M.P., Bouma,G., Metelo,J., Worth,A., Calle,Y., Cowell,L.A., Westerberg,L.S., Moulding,D.A., Mirando,S., Kinnon,C., Cory,G.O., Jones,G.E., Snapper,S.B., Burns,S.O., and Thrasher,A.J. (2009). Phosphorylation of WASp is a key regulator of activity and stability in vivo. Proc. Natl. Acad. Sci. U. S. A 106, 15738-15743. Adamovich,D.A., Nakamura,F., Worth,A., Burns,S., Thrasher,A.J., Hartwig,J.H., and Snapper,S.B. (2009). Activating mutations of N-WASP alter Shigella pathogenesis. Biochem. Biophys. Res. Commun. 384, 284-289. Ancliff,P.J., Blundell,M.P., Cory,G.O., Calle,Y., Worth,A., Kempski,H., Burns,S., Jones,G.E., Sinclair,J., Kinnon,C., Hann,I.M., Gale,R.E., Linch,D.C., and Thrasher,A.J. (2006). Two novel activating mutations in the Wiskott-Aldrich syndrome protein result in congenital neutropenia. Blood 108, 2182-2189. Chou,H.C., Anton,I.M., Holt,M.R., Curcio,C., Lanzardo,S., Worth,A., Burns,S., Thrasher,A.J., Jones,G.E., and Calle,Y. (2006). WIP regulates the stability and localization of WASP to podosomes in migrating dendritic cells. Curr. Biol. 16, 2337-2344. Frontpiece Acknowledgement Image of the tertiary structure of the interaction between WIP and the EVH1 do- main of NWASp. Reproduced with kind permision of the authors from Volkman et al., 2002 4 Contents 1 Introduction 22 2 Materials and Methods 88 3 Molecular Cloning, Protein Expression and Purification 122 4 Optimisation of Actin Polymerisation Assay 144 5 In vitro Actin Polymerisation Activity 179 6 Effect of WIP on WASp function in vitro 201 7 Effect of Cdc42 and assessment of phosphorylation on 225 WASp activation in vitro 8 EGFP-WASp expression in U937 cells 239 9 Discussion 269 Appendicies 285 References 298 5 Detailed Contents Detailed contents Frontpiece 1 Abstract 2 Acknowledgements 3 Declaration 3 Publications 4 Contents (summary) 5 Detailed contents 6 Figures 13 Tables 17 Abbreviations 18 1 Introduction 22 1.1 Clinical syndrome 22 1.1.1 Wiskott Aldrich Syndrome 22 1.1.1.1 Actin cytoskeleton in a normal immune response 23 1.1.1.2 T cell defects 25 1.1.1.3 B cell defects 26 1.1.1.4 Innate Immunity defects 27 1.1.1.5 Dendritic cell defects 28 1.1.1.6 Platelets 29 1.1.1.7 Eczema 29 1.1.1.8 Autoimmunity 29 1.1.1.9 Malignancy 30 1.1.1.10 Mouse model of Wiskott Aldrich Syndrome 30 1.1.2 X-linked Thrombocytopenia 31 1.1.3 X-linked Neutropenia 32 1.2 Genetics of WAS 33 1.2.1 WASp gene mutations 33 1.2.2 Genotype – Phenotype correlations 33 1.3 WASp family proteins 36 1.3.1 Evolution of WASp family proteins 38 1.3.2 NWASp 38 1.3.3 WAVE / SCAR proteins 38 1.4 Molecular structure of WASp 39 1.4.1 EVH1 domain 40 1.4.1.1 Proteins with homologous domains 40 1.4.1.2 Interaction with WIP and crystal structure 41 1.4.1.3 PIP2 binding 42 1.4.1.4 Src family kinase binding 42 1.4.1.5 Arp 2/3 binding and EVH1 contribution to WASp activation 42 6 Detailed Contents 1.4.1.6 Deletion of the EVH1 domain 44 1.4.2 Basic domain 44 1.4.2.1 Phospholipid binding 44 1.4.2.2 Deletion of the Basic domain 45 1.4.3 GBD and CRIB 45 1.4.3.1 Deletion of the GBD 46 1.4.4 Polyproline domain 46 1.4.4.1 Deletion of the Polyproline domain 46 1.4.5 VCA domain 48 1.4.6 Autoinhibition and Crystal structure 49 1.4.6.1 VCA – GBD autoinhibitory pseudo-domain 49 1.4.6.2 Other autoinhibitory interaction 50 1.4.6.3 Autoinhibitory conformation in vivo 50 1.4.6.4 XLN point mutations and WASp phosphorylation in GBD 51 1.5 WASp activation 51 1.5.1 Cdc-42 51 1.5.1.1 Rho Family GTPases 51 1.5.1.2 GEFs, GAPs and GDIs 53 1.5.1.3 Specificity of the Cdc-42 – WASp interaction 55 1.5.2 PIP2 56 1.5.2.1 Phosphatidylinositides in membrane dynamics 56 1.5.2.2 Cooperation with monomeric GTPases 56 1.5.2.3 Phosphatidylinositide interaction with the cytoskeleton 57 1.5.2.4 PIP2 binding to WASp 57 1.5.3 WASp activation by Cdc-42, PIP2 and TOCA 58 1.5.3.1 Experimental systems 58 1.5.3.2 Evidence of Cdc-42 induced activation 59 1.5.3.3 Crystal structure of Cdc-42 bound to WASp GBD 60 1.5.3.4 Activation of full length WASp 60 1.5.3.5 Synergistic activation by Cdc-42 and PIP2 61 1.5.3.6 TOCA1 62 1.5.3.7 Prenylation of Cdc-42 62 1.5.4 Cdc-42 independent WASp activation 63 1.5.5 Protein kinase binding and WASp phosphorylation 64 1.5.5.1 Tyrosine kinases 64 1.5.5.2 Phosphorylation of WASp 64 1.5.5.3 Role in WASp activation 66 1.5.6 SH3 domain containing proteins 67 1.5.6.1 SH3
Details
-
File Typepdf
-
Upload Time-
-
Content LanguagesEnglish
-
Upload UserAnonymous/Not logged-in
-
File Pages328 Page
-
File Size-