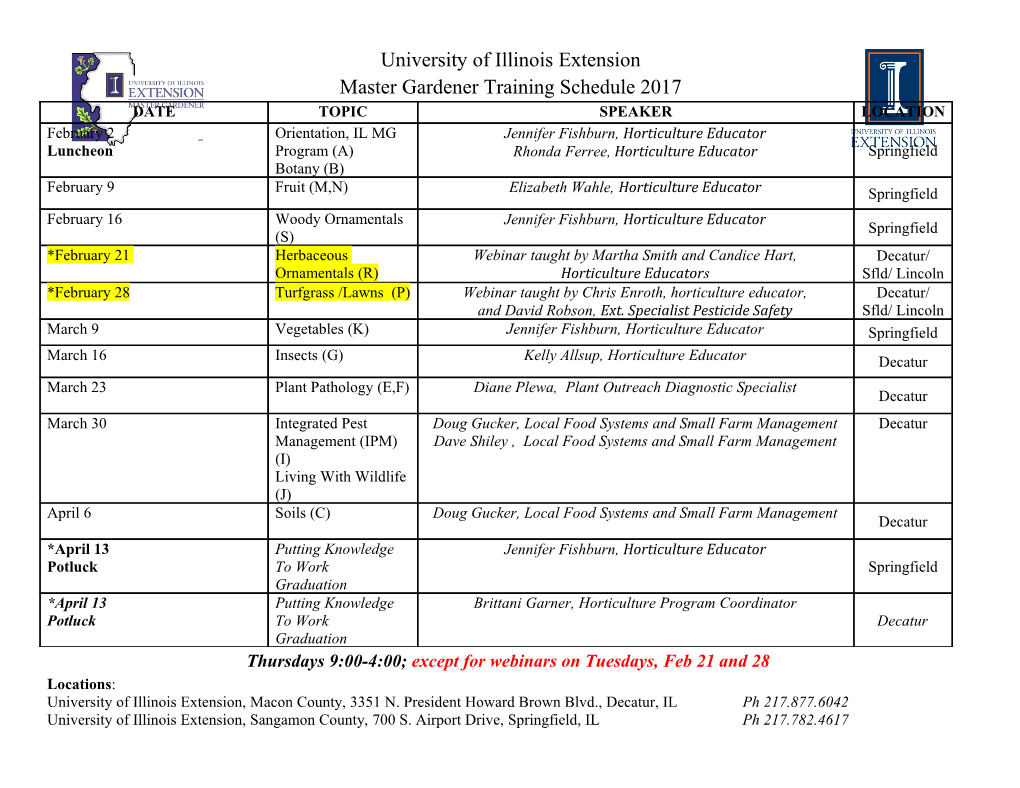
Chinese Physics C Vol. 43, No. 10 (2019) 104103 Structure and reaction dynamics of SHE Z = 130* R.R. Swain1) B. B. Sahu2) Department of Physics, School of Applied Sciences, KIIT, Deemed to be University, Bhubaneswar- 751024, Odisha, India Abstract: This study investigates the structural properties of super-heavy nuclei with Z = 130 by adopting the relativ- istic mean-field (RMF) theory within an axially deformed oscillator basis with the NL3 force parameter set. We study the binding energies, quadrupole deformation, nuclear radii, neutron separation energies, and other bulk properties. Moreover, we analyze the favorable decay modes for clear cognitive content of nuclei, such as alpha decay, using dif- ferent formulae including the Viola-Seaberg, analytical formula of Royer, universal curve formula, and universal de- cay law. We compare these with the corresponding fission process. The spontaneous fission of super-heavy nuclei is studied with Z = 130 within the mass region 310 6 A 6 340. The results exhibit good agreement with finite range droplet model (FRDM) data. This formalism presents a significant step forward in the study of the structure and de- cay modes of the isotopes of Z = 130. With this appraisal, we investigate the possible shell/sub-shell closure for su- per-heavy nuclei adjacent by decay chains of alpha and other radioactive decay particles. Keywords: relativistic mean field, nuclear bulk properties, alpha decay PACS: 21.10.Dr, 21.10.Ft, 21.10.Gv DOI: 10.1088/1674-1137/43/10/104103 1 Introduction tion with Z = 113 − 118 at JINR, Dubna [6-8]. The ele- ment Z = 113 was synthesized by a cold-fusion reaction at RIKEN, Japan [9]. In 2009 Oganessian et al. have at- Understanding the structural properties of super- tempted to synthesize the SHN with Z = 120 by the hot heavy nuclei (SHN) has become an attractive and inter- fusion reaction process [10]. However, SHN generally esting problem of nuclear physics. Heavy nuclei can be decay to known stable nuclei via α, β, and other decay formed above the uranium nuclei (Z > 92) by the nuclear reaction method. The half-life time is very small for the modes. The prediction of the theoretical model has a re- higher elements, which are yet to be observed for super- markable contribution in the search for shell/sub-shell heavy isotopes. The first heavy element that is neptuni- structure in the super-heavy region. um (Z = 93) have been evaluated at Lawrence Berkeley The theoretical model plays a crucial role in the form- = Laboratory in Berkeley (USA) [1]. These elements are ation and prediction of island of stability beyond Z 82 = mostly super-heavy elements (SHEs) that are far from the and N 126. During the last twenty years, theoretical uranium nuclei. The main objective of this study is to in- formalism has drawn attention to the formation and dis- vestigate structural properties of super-heavy nuclei. Al- tinct properties of super-heavy nuclei. All these calcula- though the theoretical model identifies island stability of tions are made to realize magic numbers beyond Z = 82, super-heavy elements [2, 3], their half-life extends from N = 126. Many theoretical calculations have been pro- minutes to years. One of the exciting challenges in this posed to expose possible shell closure, however different region is to find the stable nuclei beyond 208Pb. Currently, models give different results like FRDM, which provides the search for the magic island is an interesting topic in the magic number at Z = 114 and N = 184 [11], whereas the super-heavy region. Generally, super-heavy nuclei are the Skyrme Hartree-Fock (SHF) model concludes shell experimentally formed through hot and cold fusion reac- closure at Z = 120 or Z = 126 and N = 184 [12, 13]. This is tion processes. Some of them have been synthesized by also calculated in the study of Ref. [14] using the same cold fusion reactions with Z = 107 − 112 at GSI, Darm- RMF model, obtaining the magic nuclei at N = 172 with stadt, and RIKEN, Japan [4, 5] and the hot fusion reac- Z = 120. However, this discrepancy is related to the loca- Received 17 May 2019, Published online 29 August 2019 * Supported by project No. SR/FTP/PS-106/2013, SERB, DST, Govt. of India 1) E-mail: [email protected] 2) E-mail: [email protected] ©2019 Chinese Physical Society and the Institute of High Energy Physics of the Chinese Academy of Sciences and the Institute of Modern Physics of the Chinese Academy of Sciences and IOP Publishing Ltd 104103-1 Chinese Physics C Vol. 43, No. 10 (2019) 104103 tion of nuclei with the strongest shell effects and thus the teractions. This is also characteristic of the longest-lived longest α-decay half-lives. This is the reason that regions heavy and super-heavy nuclei. The probability of a shal- of SHEs are dominated by α-decay. The shell effects are low formation around the nucleus 342126 [37, 38] sensitive to various terms of the mean-field, such as spin- stretched towards the larger N may contain relatively orbit coupling, scalar, and effective masses. This is one of long-lived nuclei of the 128,130 as well as 127th ele- the causes for small variations in predictions of shell clos- ments necessarily close to the optimal ratio N/Z. This is ures on the effective Lagrangian used in RMF. The calcu- due to the zero electric charge of the neutron and the ab- lated proton magic numbers of SHN are quite different, sence of additional Coulomb forces destabilizing the nuc- whereas the neutron magic number is almost the same in leus. Further, the increasing survival probabilities of the every phenomenological calculation. Thus, the search for measured SHE from Z = 114 to Z = 118 seem to indicate the magic island is highly dependent on the formalism de- enhanced shell effects with increasing Z and therefore a ciding which model is to be applied. possible proton and/or neutron magic shell in the super- To find possible shell or sub-shell closure in the su- heavy region. This corresponds to the position of the su- per-heavy region, the study of structural properties along per-heavy island, in the region Z≥ 120. This is why we with the study of decay modes have equal importance to choose Z = 130 a case study in this manuscript. This ef- confirm regarding the shell stability. In this regard, spon- fort intends is to investigate the structural properties of taneous fission and the alpha decay process need to be re- the isotopes of super-heavy nuclei with Z = 130 and the vised thoroughly. Therefore, in this study, we simultan- favorable decay modes between alpha decay and fission eously investigate both the structure and decay modes of processes to understand the stability of the nuclei taking this super-heavy nuclei presented in Figs. 1–7 and Table into account the spin-orbit coupling. That is why we 1. Theoretical calculations and predictions on structures choose the RMF model for our calculation. The present and decay modes in SHN have been obtained in Refs. paper consists of four sections, Section 1 provides the in- [15-20]. As the shell closure is one of the important out- troduction, Section 2 provides the formalism that tells comes of the study of decay probability in SHN, we per- about a squat depiction of the RMF theory. In Section 3 formed a detailed study of the isotopes of SHE Z = 130. and Section 4, unique results and the conclusion are Since the shell closure exhibits some exotic behavior, presented. such as binding energy (B.E.), it may affect the fission barrier and hence the half-life [18, 21, 22]. To find the 2 Theoretical framework compatibility between the half-lives and the Q-value and hence the possible shell closure, Wang et al. [23] studied 2.1 Relativistic mean field (RMF) formalism the decay modes of SHN using 20 mass models. Among The relativistic mean-field Lagrangian density for all these models, the WS4 mass method [24] provides many-body systems can be written as [29, 30, 34, 39, 40], fine experimental results for Qα values, and the SemFIS2 µ 1 µ 1 2 2 1 3 formulae [25] gives the most accurate results for the al- L = ¯i(iγ @µ − M) i + @ σ∂µσ − mσσ − g2σ 2 2 3 pha decay half-life of super-heavy nuclei. Models such as 1 4 1 µν 1 2 µ UNIV2 formula [25], VSS [26], SP [27] and NRDX [28] − g σ − gs ¯i iσ − Ω Ωµν + m! V Vµ 4 3 4 2 formulae are in good agreement with these experimental −! −! −! −! 1 µ 2 µ 1 µν 1 2 µ results. Microscopic investigations were performed this + c3(VµV ) − g! ¯iγ i Vµ − B Bµν + mρ R :Rµ 4 4 2 study, addressing various possible decay modes for the −! µ−! µ 1 µν µ (1 − τ3i) neutron-rich SHN using the Q-value obtained from the − gρ ¯iγ τ i:R − F Fµν − e ¯iγ iAµ: 4 2 RMF model [29, 30] with the NL3 force parameter set (1) [31]. Since the RMF model has been well-accepted and Here, all the terms have their usual meanings. By applied in the study of nuclear structures regarding beta- solving the above Lagrangian equation, we can obtain the stability as well as areas in the total nuclear landscape in- field equations for both nucleons as well as mesons. cluding super-heavy nuclei [32–36], we choose this mod- Within an axially deformed harmonic oscillator basis, the el here as a tool to obtain structural properties of the SHE field equations are solved with an initial deformation Z = 130.
Details
-
File Typepdf
-
Upload Time-
-
Content LanguagesEnglish
-
Upload UserAnonymous/Not logged-in
-
File Pages8 Page
-
File Size-