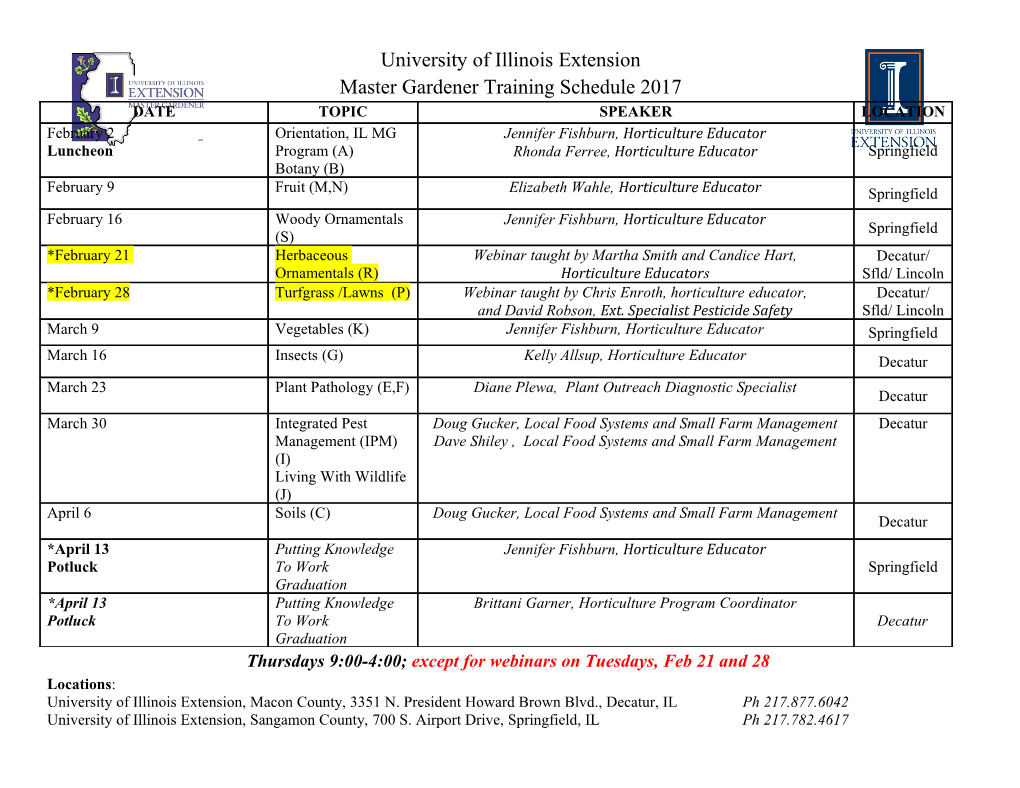
DYNAMIC CHARACTERISTICS OF MORPHING MICRO AIR VEHICLES By MUJAHID ABDULRAHIM A THESIS PRESENTED TO THE GRADUATE SCHOOL OF THE UNIVERSITY OF FLORIDA IN PARTIAL FULFILLMENT OF THE REQUIREMENTS FOR THE DEGREE OF MASTER OF SCIENCE UNIVERSITY OF FLORIDA 2004 In the name of Allah, the Gracious, the Merciful. My thesis in its entirety (apart from one sentence in the beginning of Chapter 4) is dedicated to my loving family, who have put up with my outrageous silliness in pursuit of academic achievements. To my father, who first led me down the path of innovation by helping me build my own toys. To my mother, who from the very beginning has been my advisor, counselor, and best friend. To my brother, who is my co-pilot in the clouded airspace of life. And to my sister, who is my ultimate role model for writing style and literary wit. The single outstanding sentence in Chapter 4 is dedicated to my rubber chicken, who provides irrelevant comic amusement like no other inanimate domestic animal can. Looks real, feels real, stretchable. Hells yeah. ACKNOWLEDGMENTS The work presented in this thesis was heavily supported by a large group of highly supportive people. The bulk of the mentoring, advice, suggestions, and orders came from my research advisors, Dr. Richard Lind and Dr. Peter Ifju. Dr. Lind has helped me develop an understanding of flight test objectives, modeling strategies, and, more importantly, the effect of our work on the future of aerospace. Dr. Ifju has been the ultimate source for creative inspiration in aircraft design and fabrication technique. Martin Waszak of NASA Langley Research Center has supported the UF micro air vehicle research effort for many years. In addition to providing the funding for all the research presented here, he has hosted me at LaRC for two summers on MAV design and flight testing internships. Mark Motter, also from LaRC, has provided considerable expertise in related projects. His influence carries over to the current research. Several students have also been kind enough to support the research with time, knowledge and hardware. Jason W. Grzywna and Jason Plew have provided much of the electronics hardware support for the MAVs. Jos Cocquyt, Baron Johnson, Kenneth Boothe, Shawn Mytrik, and Dan Claxton have helped extensively in solving design problems and supporting flight tests. Finally, Alfred, my rubber chicken, helped pull me through the low times when even singing ”Always Look On the Bright Side of Life” could not cheer me up. iii TABLE OF CONTENTS page ACKNOWLEDGMENTS . iii LIST OF TABLES . vi LIST OF FIGURES . vii ABSTRACT . x 1 INTRODUCTION . 1 2 BIOLOGICAL INSPIRATION . 4 3 MORPHING ON SMALL FLIGHT VEHICLES . 8 4 ASYMMETRIC WING SHAPING FOR ROLL CONTROL . 13 4.1 Aircraft Design . 13 4.2 Morphing Mechanism . 14 4.3 Flight Performance . 17 4.4 Nonlinear Modeling of Lateral and Longitudinal Dynamics . 19 5 SYMMETRIC WING TWISTING FOR ROLL CONTROL . 22 5.1 Aircraft Design . 22 5.2 Morphing Mechanism . 23 5.3 Flight Performance . 24 5.4 Linear Modeling of Lateral Dynamics . 25 5.5 Spin Characteristics of Wing Twist Morphing . 27 6 MULTI-POINT WING SHAPING . 33 6.1 Aircraft Design . 33 6.2 Morphing Mechanism . 33 6.3 Flight Performance . 35 7 VARIABLE GULL-WING ANGLE MORPHING . 37 7.1 Aircraft Design . 37 7.2 Morphing Mechanism . 38 7.3 Flight Performance . 41 7.3.1 Gliding Performance . 42 7.3.2 Climb Performance . 43 iv 7.3.3 Stall Characteristics . 44 7.4 Lateral-Directional Dynamics . 45 7.4.1 Roll Convergence . 45 7.4.2 Dutch Roll Mode . 50 7.5 Longitudinal Dynamics . 56 8 FOLDING WING AND TAIL MORPHING . 59 8.1 Aircraft Design . 59 8.2 Morphing Mechanism . 59 8.3 Flight Trials . 61 9 SUMMARY . 63 9.1 Recommendations . 63 9.2 Conclusions . 64 REFERENCES . 65 BIOGRAPHICAL SKETCH . 68 v LIST OF TABLES Table page 4–1 Properties of the 10 in and 12 in wing shaping MAVs . 14 5–1 Properties of the 24 in wing twisting MAV . 23 7–1 Wing geometry change over variable gull-wing morphing range . 38 7–2 Dutch roll modes for 0o gull-wing . 54 7–3 Dutch roll modes for 15o gull-wing . 55 7–4 Dutch roll mode eigenvectors for 0o gull-wing . 55 7–5 Dutch roll mode eigenvectors for 15o gull-wing . 56 7–6 Longitudinal modes for 0o gull-wing . 57 7–7 Longitudinal modes for 15o gull-wing . 57 8–1 Properties of the folding wing-tail aircraft in two configurations . 60 vi LIST OF FIGURES Figure page 1–1 Variable gull-wing morphing aircraft . 2 2–1 A bird alters its gull-wing angle to affect gliding angle . 5 2–2 A seagull uses differential wing extension (left) and differential wing sweep (right) . 6 2–3 A seagull extends its wings for cruising flight (left) and descends at a steep angle using gull-wing morphing (right) . 7 3–1 Micro data acquisition system . 10 3–2 Roll, pitch and yaw rate sensor board . 11 4–1 Wing shaping morphing MAVs - 10 in wingspan high-wing aircraft (left) and 12 in span mid-wing aircraft (right) . 14 4–2 Top, front, and side views of computer-aided design drawings for 12 in MAV . 15 4–3 Kevlar cables . 15 4–4 Front view showing undeflected wing (left) and morphed wing (right) . 16 4–5 Measured and predicted responses for roll rate (left), pitch rate (middle) and yaw rate (right) . 21 5–1 Wing-twisting MAV . 22 5–2 Underside view of wing showing torque rod . 23 5–3 Rear view of the 24 in MAV with undeflected (left) and morphed (right) Wing . 24 5–4 Doublet command to rudder (left), roll rate response (middle), and yaw rate response (right) . 26 5–5 Doublet command to wing twist morphing (left), roll rate response (mid- dle), and yaw rate response (right) . 27 5–6 Pilot commands (left) and responses (right) during conventional spin . 28 5–7 Pilot commands (left) and responses (right) during spin . 30 vii 5–8 Pilot commands (left) and responses (right) during cyclic spin . 31 6–1 Top, side, and front views of the 24 in span multiple-position wing shap- ing vehicle . 34 6–2 Wing shaping MAV showing neutral position (top left), wingtip morph- ing (top right), and full wing morphing (bottom) . 35 6–3 Spar torque-tube morphing actuators. The 4 front servos rotate concen- tric spar sections, aft 2 control rudder and elevator . 35 7–1 Top and side view of variable gull-wing aircraft . 38 7–2 Vehicle undergoing neutral (top), positive (center), and negative (bottom) gull-wing morphing . 39 7–3 Variable gull-wing spar structure and control linkage, linear actuator vis- ible inside fuselage at left . 40 7–4 Underside view of left wing showing wing twist effector . 41 7–5 Wing-twist command and response from flight data . 47 7–6 Pole migration with gull-wing morphing angle . 48 7–7 B-matrix value for first-order roll mode systems . 49 7–8 Wing-twist command (top) at 0o gull-wing, measured roll rate (:) and simulated roll rate (-) (bottom) . 50 7–9 Wing-twist command (top) at 15o gull-wing, measured roll rate (:) and simulated roll rate (-) (bottom) . 50 7–10 Wing-twist command (top) at 30o gull-wing, measured roll rate (:) and simulated roll rate (-) (bottom) . 51 7–11 Wing-twist command (top) at -20o gull-wing, measured roll rate (:) and simulated roll rate (-) (bottom) . 51 7–12 Rudder control pulse at 0o gull-wing angle with measured data (:) and simulated response (-) . 52 7–13 Rudder control pulse at 15o gull-wing angle with measured data (:) and simulated response (-) . 52 7–14 Open-loop Dutch roll mode pole migration for two morphing positions . 55 7–15 Frequency response diagram for 0o gull-wing (:) and 15o gull-wing (-) . 56 7–16 Elevator pulse command (left), measured (:) and simulated( -) pitch rate responses (right) . 58 viii 7–17 15o gull-wing elevator pulse command (left), measured (:) and simu- lated( -) pitch rate responses (right) . 58 8–1 Top view of unswept (left) and swept (right) configurations . 59 8–2 Side view of unswept (top) and swept (bottom) configurations . 60 8–3 Envisioned dynamic pitch up maneuver for forward to reverse flight tran- sition . 62 ix Abstract of Thesis Presented to the Graduate School of the University of Florida in Partial Fulfillment of the Requirements for the Degree of Master of Science DYNAMIC CHARACTERISTICS OF MORPHING MICRO AIR VEHICLES By Mujahid Abdulrahim December 2004 Chair: Richard Lind Major Department: Mechanical and Aerospace Engineering The research presented in this thesis is an approach to the study of flight dynamics of morphing vehicles. Case studies of several strategies are addressed in order to determine some of the basic flight characteristics of dynamically and quasi-statically morphing aircraft. These strategies include a flexible membrane wing that uses tensioned cables to shape the wing for roll control. The wing shaping for this vehicle improves roll tracking and decreases coupling compared to a rudder, even though the morphing is asymmetric. Active morphing is also implemented by using torque-rods and torque-tubes to anti-symmetrically twist a flexible wing surface. This form of morphing provided aileron-like control without a hingeline. Quasi-static morphing is used to change the gull-wing angle of an aircraft in flight. This biologically-inspired shape change alters the performance characteristics and dynamics of the vehicle and allows it to fly in several distinct flight modes. The vehicles are equipped with sensors and data logging devices and flight tested using a variety of maneuvers and techniques.
Details
-
File Typepdf
-
Upload Time-
-
Content LanguagesEnglish
-
Upload UserAnonymous/Not logged-in
-
File Pages80 Page
-
File Size-