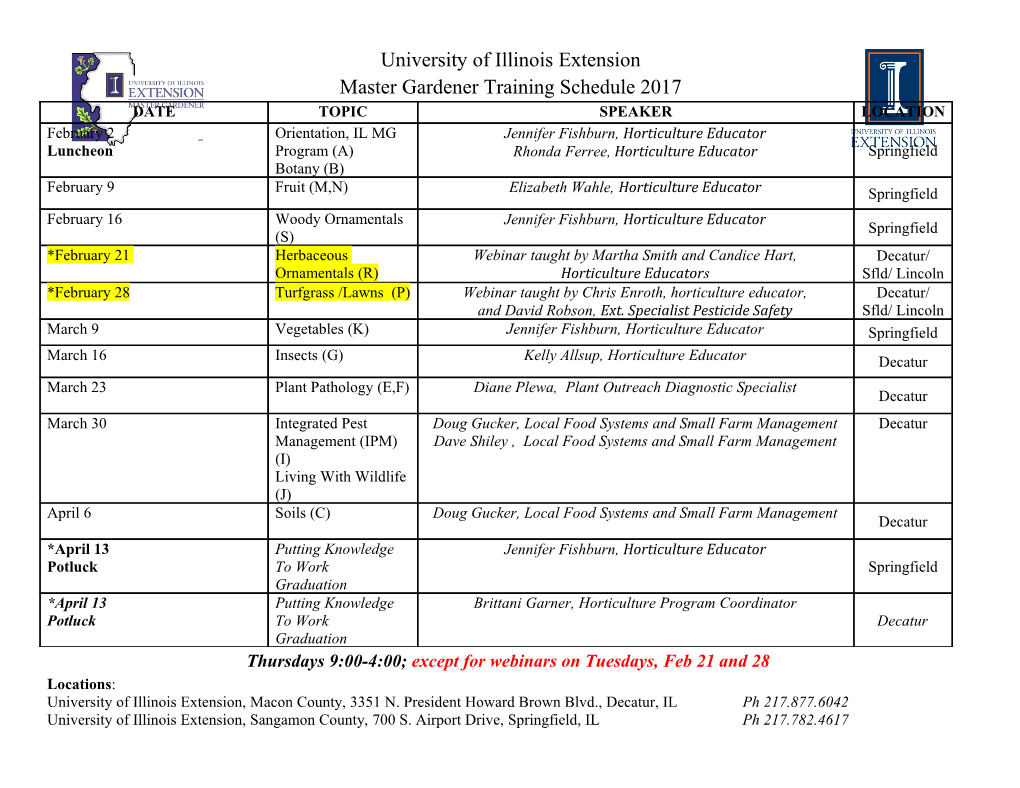
Site-Directed Mutagenesis and Ruthenium Labeling of Oxalate Decarboxylase Timothy DeMason Undergraduate Honors Thesis – Spring 2018 Department of Chemistry, University of Florida 2 Contents List of Abbreviations .................................................................................................................... 3 Abstract ......................................................................................................................................... 4 Introduction ................................................................................................................................... 5 Methods......................................................................................................................................... 11 Results and Discussion ................................................................................................................. 16 Calculation of Ru—Mn Electron Transfer ....................................................................... 16 Mutagenesis of K375C/C383A ......................................................................................... 18 Synthesis of Ru-OxDC ..................................................................................................... 22 Conclusion .................................................................................................................................... 25 Acknowledgements ....................................................................................................................... 26 References ..................................................................................................................................... 26 3 List of Abbreviations bis-tris 2-bis(2-hydroxyethyl)amino-2-(hydroxymethyl)-1,3-propanediol bpy 2,2’-bipyridine DMSO dimethyl sulfoxide DTT dithiothreitol E. coli Escherichia coli FDH Formate Dehydrogenase IA-phen 5-iodoacetamido-1,10-phenanthroline IPTG isopropyl β-D-1-thiogalactopyranoside LRET Long Range Electron Transfer mM mili Molar μM micro Molar MS Mass Spectrometry NAD+ nicotinamide adenine dinucleotide OxDC Oxalate Decarboxylase PCET Proton Coupled Electron Transfer PCR Polymerase Chain Reaction PDB Protein Data Bank Ru-OxDC Ruthenium Modified Oxalate Decarboxylase Tris 2-amino-2-(hydroxymethyl)-1,3-propanediol WT Wild Type Oxalate Decarboxylase 4 Abstract Oxalate is a toxic dicarboxylic acid that is decomposed into carbon dioxide and formate by Bacillus subtilis Oxalate Decarboxylase (OxDC). Each monomer of OxDC contains two manganese ions, one at the N-terminal end and one at the C-terminal end. Evidence suggests that the C-terminal manganese plays a functional role in the mechanism of catalysis, which can be further investigated by studying a ruthenium labeled OxDC. A K375C/C383A OxDC mutant was generated, which places a cysteine close to the C-terminal manganese for labeling with the 2+ thiol reactive ruthenium compound [Ru(bpy)2(IA-phen)] . Marcus theory calculations predict a tunneling time of 660 ns between the ruthenium and C-terminal manganese ions. The K375C/C383A OxDC mutant displayed typical wild type Michaelis-Menten kinetics, -1 with KM = 10 ± 2 mM and kcat = 90 ± 13 s . Attempts at labeling K375C/C383A OxDC with 2+ [Ru(bpy)2(IA-phen)] were unsuccessful. The failure of the labeling reaction appears to be due 2+ to an inability of [Ru(bpy)2(IA-phen)] to react with K375C/C383A OxDC rather than nonspecificity of the reaction. Future labeling with other thiol reactive ligands should be attempted. 5 Introduction Oxalic acid is a dicarboxylic acid with pKa’s of 1.2 and 4.2.1 Oxalic acid is produced in plants through several biochemical pathways including the activity of glyoxalate oxidase and isocitrate lysase.2,3 Oxalate is known to precipitate in the presence of divalent cations.1 Calcium oxalate is one of the more important salts since it is relatively insoluble and is present in about 60% of kidney stones.4 Developing ways of reducing the degree of oxalate accumulation in the kidneys is of medical importance. The buildup of calcium oxalate deposits can also cause problems in the manufacturing of paper.5,6 Oxalate Decarboxylase (OxDC) is an oxalate degrading enzyme found in Bacillus subtilis and other organisms. The enzyme catalyzes the degradation of oxalate into formate and carbon dioxide in 99.8% of turnovers (decarboxylase pathway), and two equivalents of carbon dioxide and hydrogen peroxide in the rest (oxidase pathway), as shown in Schemes 1A and 1B.1 – O O O O2 A CO2 + – O OH H O – O O B + O H 2 CO H O + 2 + 2 + 2 2 O OH Scheme 1: A) Degradation of oxalate into carbon dioxide and formate and B) of oxalate into carbon dioxide and hydrogen peroxide. Native OxDC exists as a homo-hexamer formed from a dimer of trimers (see Figure 1C). The structure of the monomer is shown in Figures 1A and 1B and consists of two β-barrel domains, one at the N-terminal end (shown in green in Figures 1A and 1B) and another at the C- 6 terminal end (shown in blue in Figures 1A and 1B). Inside each β-barrel rests a manganese ion bound to three histidines and one glutamate, leaving two sites free for other small ligands. A) C) B) Figure 1: The crystal structure of Bacillus subtilis OxDC showing the manganese ions in purple. A) Monomer structure as viewed from the side. B) Monomer viewed from the top. C) Hexamer viewed from the top with one trimer in blue and the other in orange. These figures were generated in PyMOL using the 1UW8 PDB file. The mechanism of OxDC catalysis of oxalate is still not completely understood. Figure 2 illustrates the current proposed mechanism for catalysis. First, mono-protonated oxalate and dioxygen bind to the N-terminal manganese, with the dioxygen generating Mn3+.1 Then, glutamate-162 removes the remaining acidic proton from oxalate, while simultaneously an electron is transferred from oxalate to the manganese, i.e. proton coupled electron transfer (PCET).1 Heterolytic cleavage of the oxalate carbon-carbon bond follows, liberating carbon dioxide and producing a carbon dioxide radical anion still bound to the manganese.1 Finally, PCET occurs again, reducing the carbon dioxide radical anion while oxidizing the Mn2+ to 7 regenerate Mn3+. Concurrently, glutamate-162 protonates the carbon of the carbon dioxide radical anion to form formate.1 A problem with this mechanism is that it places a dioxygen radical and carbon dioxide radical anion in close proximity. These two radicals are expected to react to form - peroxycarbonate (HCO4 ) which may then decompose with proton uptake to produce hydrogen peroxide and carbon dioxide as is the case in the oxidase pathway.7 However, the products of the oxidase pathway are only observed in 0.2% of turnovers.1 Figure 2: Current proposed mechanism for OxDC. The N-terminal manganese binds oxalate (and oxygen as a co-catalyst) while it cycles between Mn2+ and Mn3+. From (9) with permission from Elsevier. While the C-terminal manganese was originally thought to only play a structural role, further investigation into the OxDC mechanism suggests that the C-terminal manganese may play a role in catalysis. The presence of a stacked tryptophan dimer between the N- and C- terminal manganese of adjacent monomers suggests that electron transfer between the two manganese ions is possible. Substitution of tryptophan-96 and tryptophan-274 with 8 phenylalanine or tyrosine leads to significantly reduced catalytic capability indicating their importance in catalysis.1,8 Additionally, EPR spin trapping studies of a flexible lid mutant suggest that the carbon dioxide radical anions and superoxide radicals are produced in separate locations.9 Since oxalate is known to bind at the N-terminal manganese, perhaps oxygen binds to the C-terminal manganese.1 A new mechanism for OxDC catalysis is shown in Figure 3, proposing the C-terminal manganese site as the temporary electron sink through the use of long range electron transfer (LRET).1010 C-terminal N-terminal O O Glu Glu Glu Glu 280 101 280 101 – His + HC O - O O His 319 O 140 OH2 2 4 His 319 O His 140 2+ 2+ 2+ 2+ Mn Mn Mn Mn His 275 OH2 His 97 OH2 His 275 OH2 His 97 O OH O His O His 273 His 95 His 273 95 – – Glu O Glu162 O 162 LRET + H+ OH OH Glu Glu Glu Glu 280 101 – 280 101 – O PCET O O His 319 O His 140 O His 319 O His 140 2+ 2+ oxidation 2+ 3+ Mn Mn + Mn Mn C – His 275 OH2 His 97 O O His 275 OH2 His 97 O OH His O His His 273 95 His 273 95 O HO Glu162 – O Glu162 - - CO2 - CHOO - + HC2O4 OH OH Glu Glu Glu 280 101 PCET 280 Glu101 His His 319 O 140 reduction His 319 O His 140 2+ 2+ 2+ 3+ H Mn Mn – Mn Mn C His His – 275 OH2 97 O O His 275 OH2 His 97 O O His His His 273 95 O His 273 95 O – HO Glu162 O Glu162 Figure 3: New proposed mechanism for OxDC, including the possibility of long range electron transfer, assuming oxalate binds in a bi-dentate fashion. From (10). 9 Selective oxidation of the C-terminal manganese could be used to further study the possibility of LRET. Ruthenium modified proteins have been used previously to study the electron transfer properties of metalloproteins such as azurin, cytochrome c, and myoglobin.11 These modified proteins employ the use of the so-called “flash-quench” technique shown in Scheme 2A. A ruthenium(II) diimine complex, such as tris(bipyridine)ruthenium(II), is excited with a “flash” of light at 450 nm to produce an excited state which is then “quenched” by either an oxidant or reductant to produce ruthenium(III) or ruthenium(I), respectively.12 The oxidized or reduced ruthenium can then either remove or inject
Details
-
File Typepdf
-
Upload Time-
-
Content LanguagesEnglish
-
Upload UserAnonymous/Not logged-in
-
File Pages28 Page
-
File Size-