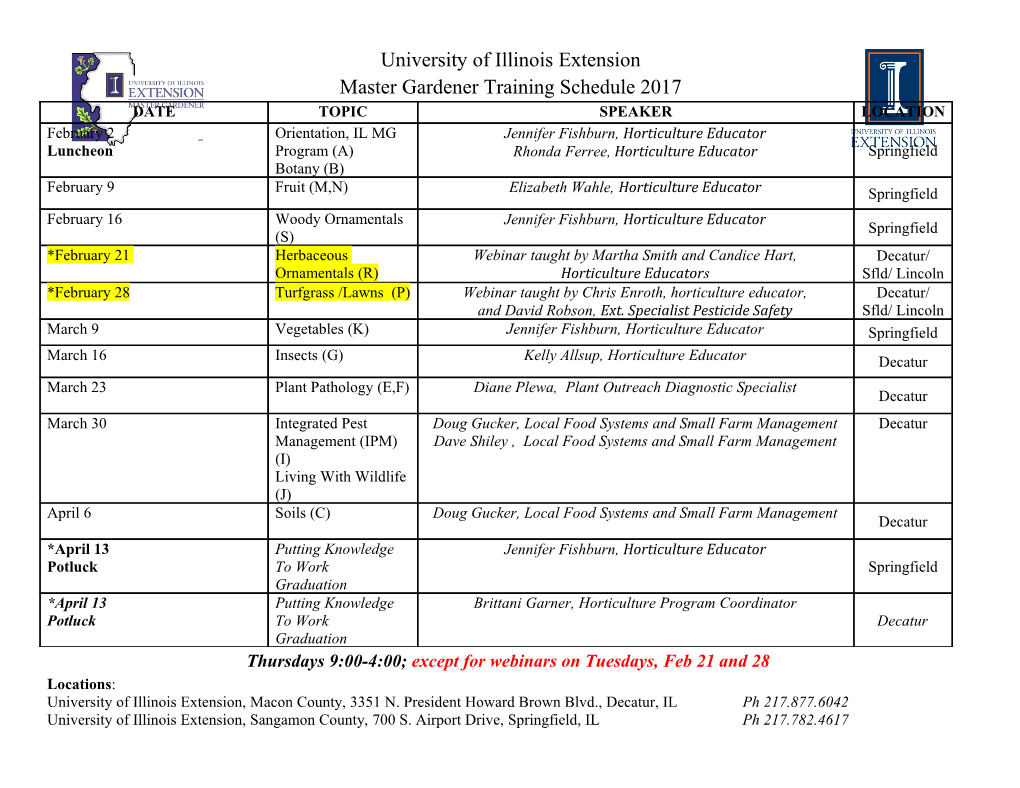
The missing link in gravitational-wave astronomy: Discoveries waiting in the decihertz range Manuel Arca Sedda1, Christopher P L Berry2;3, Karan Jani4, Pau Amaro-Seoane5;6;7;8, Pierre Auclair9, Jonathon Baird10, Tessa Baker11, Emanuele Berti12, Katelyn Breivik13, Adam Burrows14, Chiara Caprini9, Xian Chen15;6, Daniela Doneva16, Jose M Ezquiaga17, K E Saavik Ford18, Michael L Katz2, Shimon Kolkowitz19, Barry McKernan18, Guido Mueller20, Germano Nardini21, Igor Pikovski22, Surjeet Rajendran12, Alberto Sesana23, Lijing Shao6, Nicola Tamanini24, David Vartanyan25, Niels Warburton26, Helvi Witek27, Kaze Wong12, Michael Zevin2 1 Astronomisches Rechen-Institut, Zentr¨umf¨urAstronomie, Universit¨atHeidelberg, M¨onchofstr. 12-14, Heidelberg, Germany 2 Center for Interdisciplinary Exploration and Research in Astrophysics (CIERA), Department of Physics and Astronomy, Northwestern University, 1800 Sherman Avenue, Evanston, IL 60201, USA 3 SUPA, School of Physics and Astronomy, University of Glasgow, Glasgow G12 8QQ, UK 4 Department of Physics and Astronomy, Vanderbilt University, Nashville, TN 37212, USA 5 Universitat Polit`ecnicade Val`encia,IGIC, Spain 6 Kavli Institute for Astronomy and Astrophysics, Peking University, Beijing 100871, China 7 Institute of Applied Mathematics, Academy of Mathematics and Systems Science, CAS, Beijing 100190, China 8 Zentrum f¨ur Astronomie und Astrophysik, TU Berlin, Hardenbergstraße 36, 10623 arXiv:1908.11375v3 [gr-qc] 27 Jul 2020 Berlin, Germany 9 Laboratoire Astroparticule et Cosmologie, CNRS UMR 7164, Universit´e Paris-Diderot, 10 rue Alice Domon et L´eonieDuquet, 75013 Paris, France 10 High Energy Physics Group, Physics Department, Imperial College London, Blackett Laboratory, Prince Consort Road, London, SW7 2BW, UK 11 School of Physics and Astronomy, Queen Mary University of London, Mile End Road, London, E1 4NS, UK 12 Department of Physics and Astronomy, Johns Hopkins University, 3400 N. Charles Street, Baltimore, MD 21218, USA 13 Canadian Institute for Theoretical Astrophysics, University of Toronto, 60 St. George Street, Toronto, Ontario, M5S 1A7, Canada 14 Department of Astrophysical Sciences, University of Princeton, 4 Ivy Lane, Princeton, NJ 08544-1001, US 15 Astronomy Department, School of Physics, Peking University, Beijing 100871, China The missing link in GW astronomy 2 16 Theoretical Astrophysics, Eberhard Karls University of T¨ubingen,T¨ubingen 72076, Germany 17 Kavli Institute for Cosmological Physics and Enrico Fermi Institute, The University of Chicago, Chicago, IL 60637, USA 18 City University of New York-BMCC, 199 Chambers St, New York, NY 10007, USA & Dept. of Astrophysics, American Museum of Natural History, New York, NY 10028, USA 19 Department of Physics, University of Wisconsin { Madison, Madison, WI 53706, USA 20 Department of Physics, University of Florida, PO Box 118440, Gainesville, Florida 32611, USA 21 Faculty of Science and Technology, University of Stavanger, 4036 Stavanger, Norway 22 Department of Physics, Stevens Institute of Technology, Hoboken, NJ 07030, USA & The Oskar Klein Centre, Department of Physics, Stockholm University, Stockholm, Sweden 23 Universit`adi Milano Bicocca, Dipartimento di Fisica G. Occhialini, Piazza della Scienza 3, I-20126, Milano, Italy 24 Max-Planck-Institut f¨urGravitationsphysik (Albert-Einstein-Institut), Am M¨uhlenberg 1, 14476 Potsdam-Golm, Germany 25 Department of Astronomy (University of California), Berkeley, CA 94720-3411, USA 26 School of Mathematics and Statistics, University College Dublin, Belfield, Dublin 4, Ireland 27 Department of Physics, King's College London, Strand, London, WC2R 2LS, United Kingdom E-mail: [email protected], [email protected], [email protected] 29 July 2020 Abstract. The gravitational-wave astronomical revolution began in 2015 with LIGO's observation of the coalescence of two stellar-mass black holes. Over the coming decades, ground-based detectors like LIGO, Virgo and KAGRA will extend their reach, discovering thousands of stellar-mass binaries. In the 2030s, the space- based LISA will enable gravitational-wave observations of the massive black holes in galactic centres. Between ground-based observatories and LISA lies the unexplored decihertz gravitational-wave frequency band. Here, we show the potential of a Decihertz Observatory which could cover this band, and complement discoveries made by other gravitational-wave observatories. The decihertz range is uniquely suited to 2 4 observation of intermediate-mass ( 10 {10 M ) black holes, which may form the ∼ missing link between stellar-mass and massive black holes, offering an opportunity to measure their properties. Decihertz observations will be able to detect stellar- mass binaries days to years before they merge and are observed by ground-based detectors, providing early warning of nearby binary neutron star mergers, and enabling measurements of the eccentricity of binary black holes, providing revealing insights into their formation. Observing decihertz gravitational-waves also opens the possibility of testing fundamental physics in a new laboratory, permitting unique tests of general relativity and the Standard Model of particle physics. Overall, a Decihertz Observatory would answer outstanding questions about how black holes form and evolve across The missing link in GW astronomy 3 cosmic time, open new avenues for multimessenger astronomy, and advance our understanding of gravitation, particle physics and cosmology. Keywords: Gravitational-wave detectors, Decihertz Observatories, compact binaries, multiband gravitational-wave astronomy, intermediate-mass black holes, tests of general relativity, early Universe physics Submitted to: Class. Quantum Grav. 1. The astronomical revolution During the 20th century there was an explosion of astronomical discoveries as new instruments enabled us to observe more of the electromagnetic spectrum [1]. Diversifying out from visible light provided a richer understanding of the cosmos and provided many unexpected discoveries|from radio pulsars [2, 3] to gamma-ray bursts [4{6]. A similar revolution awaits gravitational-wave (GW) astronomy [7, 8], and we discuss the scientific potential of exploring the 0:01{1 Hz GW spectrum. ∼ On 14 September 2015 the twin Laser Interferometer Gravitational-Wave Observatory (LIGO) detectors made the first observation of a GW signal [8]. GW150914 originated from a coalescence of two black holes (BHs) each about 30M [9, 10]. This discovery enabled revolutionary advances in the understanding of the astrophysics of binary BHs (BBHs) [11, 12] and the nature of gravity [13, 14]. The signal was observed sweeping through a frequency range of 20{250 Hz. The lower frequency limit is set ∼ by the sensitivity of the detectors, because seismic noise prevents observations at low frequencies. The upper limit is set by the merger frequency of the BHs, which is inversely proportional to the binary's total mass. Ground-based detectors, like LIGO [15], Virgo [16] and KAGRA [17], can observe across a range of frequencies 10{103 Hz. ∼ This is well tailored to the detection of merging stellar-mass BH and neutron star (NS) binaries [10, 18{20], but it is only a small part of the GW spectrum. Next-generation ground-based detectors, like Cosmic Explorer [21] or the Einstein Telescope [22] may extend the observable range of GW frequencies down to a few hertz, but pushing lower requires switching to space-based observatories. Observing at lower GW frequencies enables study of the mergers of more massive binaries, and measurements of stellar-mass binaries earlier in their inspirals. The space- based Laser Interferometer Space Antenna (LISA), due for launch in 2034, will observe across frequencies 10−4{10−1 Hz, being most sensitive around 3 10−3 Hz [23]. This ∼ × 6 makes it perfectly suited to observe the merger of binaries with 10 M massive ∼ BHs [24{26]. These massive BHs are found in the centres of galaxies [27, 28], including our own Milky Way [29]. LISA would also have been able to observe a binary like GW150914's source years{days prior to merger [30]. Making multiband observations of The missing link in GW astronomy 4 stellar-mass binaries opens up new avenues of investigation, including unravelling how the systems formed [31, 32] and enabling precision tests of gravity [33, 34]. At even lower frequencies, pulsar timing arrays are sensitive to GWs of 10−9{ −7 9 ∼ 10 Hz [35]. This makes them well suited to observe 10 M supermassive BHs [36]. ∼ How (super)massive BHs form and evolve is currently an active area of research with many outstanding questions. Combining observations from LISA and pulsar timing will provide a unique insight into the growth of supermassive BHs [37, 38]. Here, we explore the case for extending the accessible GW spectrum with an observatory that can observe in the 0:01{1 Hz decihertz range. Such observations ∼ would (i) unravel the channels driving the formation of stellar-mass binaries, enhancing ground-based observations with deeper multiband observations compared to those achievable with LISA; (ii) complete our picture of the population of BHs by providing unrivaled measurements of intermediate-mass BHs (IMBHs), which may be the missing link in the formation and evolution of (super)massive BHs, and (iii) enable tests of fundamental physics in a new regime. A decihertz GW observatory has the unrivaled potential to answer questions about the complicated physical processes that regulate binary star formation and evolution, to examine the
Details
-
File Typepdf
-
Upload Time-
-
Content LanguagesEnglish
-
Upload UserAnonymous/Not logged-in
-
File Pages52 Page
-
File Size-