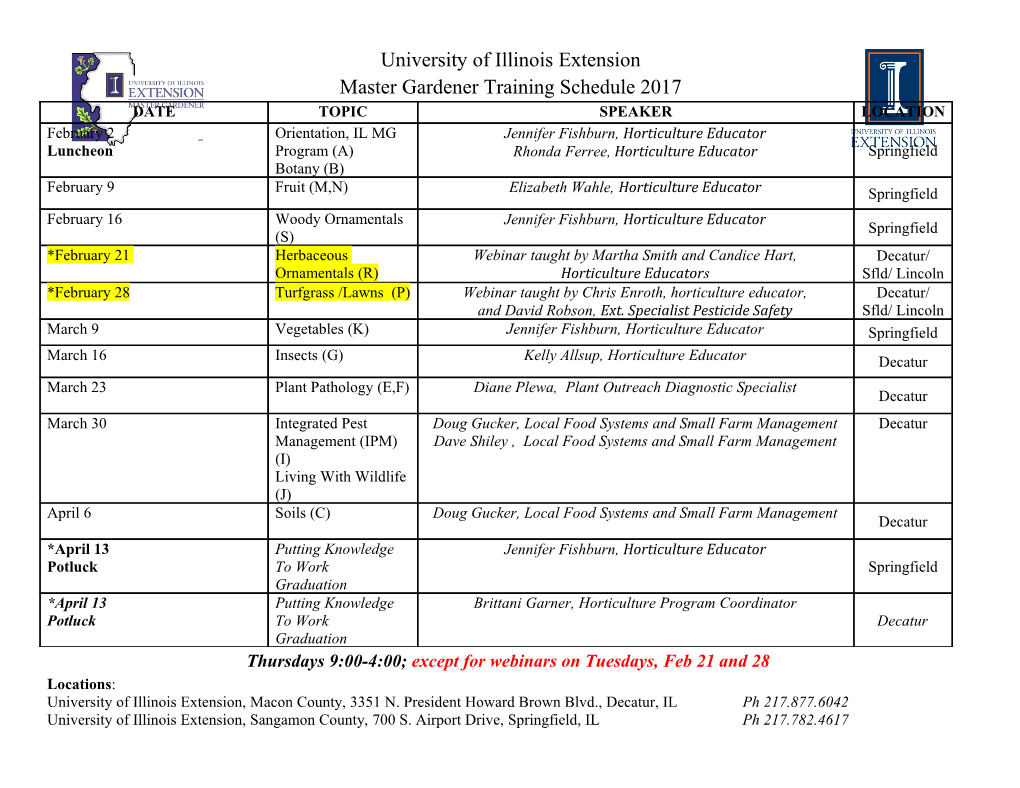
Biogeosciences, 15, 6997–7024, 2018 https://doi.org/10.5194/bg-15-6997-2018 © Author(s) 2018. This work is distributed under the Creative Commons Attribution 4.0 License. Factors controlling coccolithophore biogeography in the Southern Ocean Cara Nissen, Meike Vogt, Matthias Münnich, Nicolas Gruber, and F. Alexander Haumann Institute for Biogeochemistry and Pollutant Dynamics, ETH Zürich, Universitätstrasse 16, 8092 Zürich, Switzerland Correspondence: Cara Nissen ([email protected]) Received: 29 March 2018 – Discussion started: 6 April 2018 Revised: 8 November 2018 – Accepted: 9 November 2018 – Published: 22 November 2018 Abstract. The biogeography of Southern Ocean phytoplank- 1 Introduction ton controls the local biogeochemistry and the export of macronutrients to lower latitudes and depth. Of particu- The ocean is changing at an unprecedented rate as a con- lar relevance is the competitive interaction between coc- sequence of increasing anthropogenic CO2 emissions and colithophores and diatoms, with the former being prevalent related climate change. Changes in density stratification along the “Great Calcite Belt” (40–60◦ S), while diatoms and nutrient supply, as well as ocean acidification, lead to tend to dominate the regions south of 60◦ S. To address the changes in phytoplankton community composition and con- factors controlling coccolithophore distribution and the com- sequently ecosystem structure and function. Some of these petition between them and diatoms, we use a regional high- changes are already observable today (e.g., Soppa et al., resolution model (ROMS–BEC) for the Southern Ocean (24– 2016; Winter et al., 2013) and may have cascading effects 78◦ S) that has been extended to include an explicit repre- on global biogeochemical cycles and oceanic carbon uptake sentation of coccolithophores. We assess the relative impor- (Laufkötter et al., 2016; Freeman and Lovenduski, 2015; tance of bottom-up (temperature, nutrients, light) and top- Cermeño et al., 2008). Changes in Southern Ocean (SO) bio- down (grazing by zooplankton) factors in controlling South- geography are especially critical due to the importance of the ern Ocean coccolithophore biogeography over the course of SO in fueling primary production at lower latitudes through the growing season. In our simulations, coccolithophores are the lateral export of nutrients (Sarmiento et al., 2004) and an important member of the Southern Ocean phytoplankton in taking up anthropogenic CO2 (Frölicher et al., 2015). For community, contributing 17 % to annually integrated net pri- the carbon cycle, the ratio of calcifying and noncalcifying mary productivity south of 30◦ S. Coccolithophore biomass phytoplankton is crucial due to the counteracting effects of ◦ is highest north of 50 S in late austral summer, when light calcification and photosynthesis on seawater pCO2, which levels are high and diatoms become limited by silicic acid. ultimately controls CO2 exchange with the atmosphere, and Furthermore, we find top-down factors to be a major control the differing ballasting effect of calcite and silicic acid shells on the relative abundance of diatoms and coccolithophores for organic carbon export. in the Southern Ocean. Consequently, when assessing poten- Calcifying coccolithophores and silicifying diatoms tial future changes in Southern Ocean coccolithophore abun- are globally ubiquitous phytoplankton functional groups dance, both abiotic (temperature, light, and nutrients) and bi- (O’Brien et al., 2013; Leblanc et al., 2012). Diatoms are a otic factors (interaction with diatoms and zooplankton) need major contributor to global phytoplankton biomass (≈ 6 %– to be considered. 70 %; Buitenhuis et al., 2013b) and annual net primary pro- duction (40 % of NPP; Sarthou et al., 2005). In compari- son, coccolithophores contribute less to biomass (≈ 0:04 %– 6 %; Buitenhuis et al., 2013b) and to global NPP (0.4 %– 17 %, model-derived estimates using a variety of coccol- ithophore parameterizations; see O’Brien, 2015; Jin et al., 2006; Moore et al., 2004; Gregg and Casey, 2007a). How- Published by Copernicus Publications on behalf of the European Geosciences Union. 6998 C. Nissen et al.: Southern Ocean coccolithophore biogeography ever, coccolithophores are the major phytoplanktonic calci- lite data from the North Atlantic, Behrenfeld(2014) stresses fier (Iglesias-Rodríguez et al., 2002), thereby significantly the importance of simultaneously considering bottom-up and impacting the global carbon cycle. Diatoms dominate the top-down factors when assessing seasonal phytoplankton phytoplankton community in the SO (e.g., Trull et al., 2018; biomass dynamics and the succession of different phyto- Swan et al., 2016; Wright et al., 2010), but coccolithophores plankton types owing to the spatially and temporally vary- have received increasing attention in recent years. Satel- ing relative importance of the physical–biogeochemical and lite imagery of particulate inorganic carbon (PIC, a proxy the biological environment. In the SO, previous studies have for coccolithophore abundance) revealed the “Great Cal- shown zooplankton grazing to control total phytoplankton cite Belt” (GCB; Balch et al., 2011), an annually reoccur- biomass (Le Quéré et al., 2016), phytoplankton community ring circumpolar band of elevated PIC concentrations be- composition (Scotia Weddell Sea; Granéli et al., 1993), and tween 40 and 60◦ S. In situ observations confirmed coc- ecosystem structure (Smetacek et al., 2004; De Baar, 2005), colithophore abundances of up to 2:4 × 103 cells mL−1 in suggesting that top-down control might also be an important the Atlantic sector (blooms on the Patagonian Shelf), up to driver for the relative abundance of coccolithophores and di- 3:8×102 cells mL−1 in the Indian sector (Balch et al., 2016), atoms. But the role of zooplankton grazing in current Earth and up to 5:4×102 cells mL−1 in the Pacific sector of the SO system models is not well considered (Sailley et al., 2013; (Cubillos et al., 2007) with Emiliania huxleyi being the dom- Hashioka et al., 2013), and the impact of different grazing inant species (Balch et al., 2016; Saavedra-Pellitero et al., formulations on phytoplankton biogeography and diversity is 2014). However, the contribution of coccolithophores to to- subject to ongoing research (e.g., Prowe et al., 2012; Vallina tal SO phytoplankton biomass and NPP has not yet been as- et al., 2014). sessed. Locally, elevated coccolithophore abundance in the While none of the SO in situ studies directly assessed in- GCB has been found to turn surface waters into a source of teractions of diatoms and coccolithophores over the course of CO2 for the atmosphere (Balch et al., 2016), emphasizing the the year, some in situ studies infer a diatom–coccolithophore necessity to understand the controls on their abundance in the succession from depleted silicic acid coinciding with iron SO in the context of the carbon cycle and climate change. levels high enough to sustain elevated coccolithophore abun- While coccolithophores have been observed to have moved dance (high Fe, low Si niche; Balch et al., 2016, 2014; Painter polewards in recent decades (Rivero-Calle et al., 2015; Win- et al., 2010). In contrast to this, recent in situ and satellite ter et al., 2013; Beaugrand et al., 2012), their response to the studies find coccolithophores and diatoms to coexist rather combined effects of future warming and ocean acidification than succeed each other throughout the growth season in the is still subject to debate (Schlüter et al., 2014; Beaugrand North Atlantic (Daniels et al., 2015) and the global open et al., 2012; Beaufort et al., 2011; Iglesias-Rodríguez et al., ocean (Hopkins et al., 2015). In fact, large areas of the GCB 2008; Riebesell et al., 2000). As their response will also cru- have been identified as “coexistence” areas (Hopkins et al., cially depend on future phytoplankton community composi- 2015), thereby putting into question the succession pattern tion and predator–prey interactions (Dutkiewicz et al., 2015), predicted by Margalef’s mandala (Margalef, 1978) and re- it is essential to assess the controls on their abundance in to- sults of in situ studies for the SO (Balch et al., 2016, 2014; day’s climate. Painter et al., 2010). This highlights the necessity to better Coccolithophore biomass is controlled by a combination understand the drivers and seasonal dynamics of the relative of bottom-up (physical–biogeochemical environment) and importance of coccolithophores and diatoms in the SO before top-down factors (predator–prey interactions), but the rela- assessing potential future changes. tive importance of the two has not yet been assessed for coc- In this study, we use a regional high-resolution model for colithophores in the SO. Bottom-up factors directly impact the SO to simultaneously assess the relative importance of phytoplankton growth, and diatoms and coccolithophores are bottom-up versus top-down factors in controlling SO coc- traditionally discriminated based on their differing require- colithophore biogeography over a complete annual cycle. In ments for nutrients, turbulence, and light. Based on this, Mar- particular, we assess the role of diatoms in constraining high galef’s mandala predicts a seasonal succession from diatoms coccolithophore abundance and the importance of microzoo- to coccolithophores as light levels increase and nutrient lev- plankton and macrozooplankton grazing for the relative im- els decline (Margalef, 1978). In situ studies assessing SO portance
Details
-
File Typepdf
-
Upload Time-
-
Content LanguagesEnglish
-
Upload UserAnonymous/Not logged-in
-
File Pages28 Page
-
File Size-