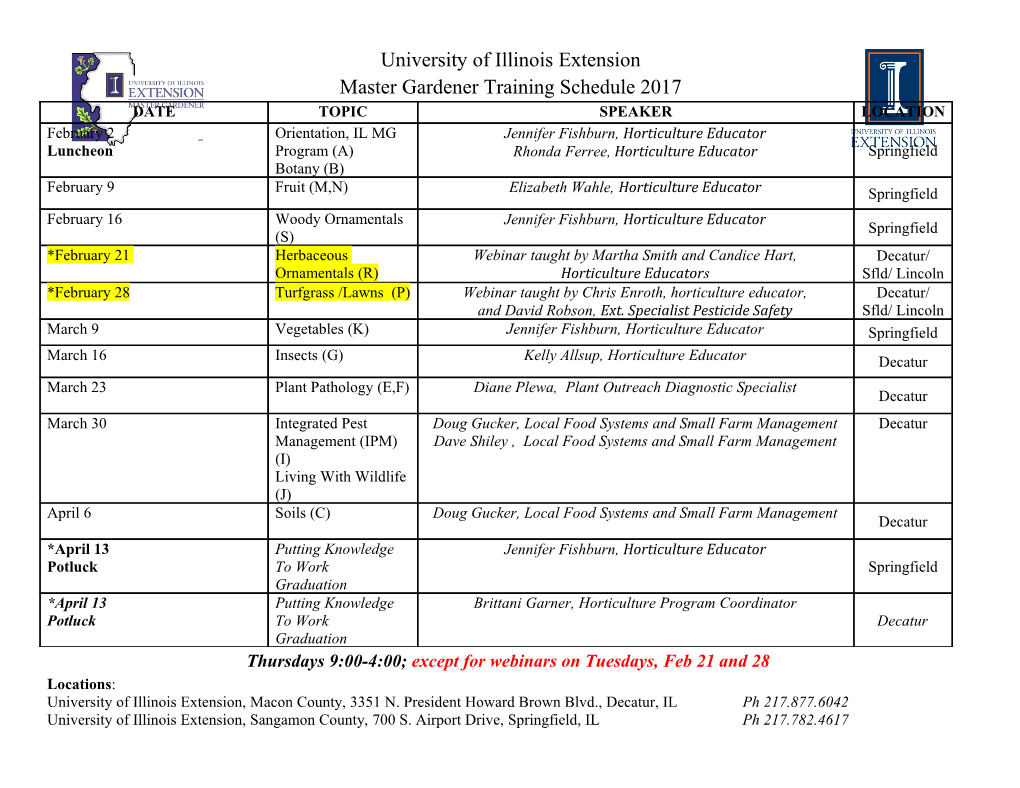
Neutron Monitor Resp onse Functions J.M. Clem Bartol Research Institute, University of Delaware, Newark, DE, USA L.I. Dorman IZMIRAN, Moscow, Russia; Technion, Haifa, and Israel Cosmic Ray Center, aliated with Tel Aviv University Abstract. The neutron monitor provides continuous ground-based recording of the hadronic comp onent in atmospheric secondary radiation which is related to primary cos- mic rays. Simpson 1948 discovered that the latitude variation of the secondary hadronic comp onentwas considerably larger than the muon comp onent suggesting the resp onse of a neutron monitor is more sensitivetolower energies in the pri- mary sp ectrum. The di erent metho ds of determining the neutron monitor resp onse function of primary cosmic rays are reviewed and discussed including early and recent results. The authors also provide results from a new calculation Clem, 1999 including angle dep endent yield functions for di erent neutron monitor typ es which are calculated using a simulation of cosmic ray air showers combined with a detection eciency simulation for di erent secondary particle sp ecies. Results are shown for 10 3 IGY and NM64 con gurations using the standard BF detectors and the new He 3 detectors to b e used in the Spaceship Earth Pro ject Bieb er et al., 1995. The metho d of calculation is describ ed in detail and the results are compared with measurements and previous calculation s. A summary of future goals is discussed. Keywords: Neutron Monitor, Resp onse Function, Cosmic Rays 1. Intro duction In order to understand the ground-based neutron monitor as a primary particle detector a relationship b etween the count rate and primary ux must b e established. Primary particles, not rejected by the geomagnetic eld, enter the atmosphere and undergo multiple interactions resulting in showers of secondary particles whichmay reach ground level and b e detected by a neutron monitor. Therefore a yield function must in- corp orate the propagation of particles through the Earth's atmosphere and the detection resp onse of a neutron monitor to secondary particles such as neutrons, protons, muons and pions. The resp onse functions can then b e determined by convolving the cosmic ray sp ectra with the yield function. The exp ected count rate from latitude surveys can b e calculated byintegrating the resulting resp onse functions. After the invention of the neutron monitor by Simpson 1948, the concept of resp onse functions was intro duced byFonger 1953, Brown c 2000 Kluwer Academic Publishers. Printed in the Netherlands. issi_final6.tex; 14/01/2000; 12:50; p.1 2 Figure1. Count rate recorded on the Italian Antarctic Program 3-NM-64 survey during 1996-97 Villoresi, 1997. 1957 and Dorman 1957 , and since then, a number of ventures have b een taken to fully characterize these functions and to understand their inherent features. Complete interpretation requires knowledge of the detection eciency, atmospheric particle transp ort, primary comp o- sition and energy sp ectrum, and geomagnetic particle transp ort. This pap er fo cuses sp eci cally on particle transp ort through the atmosphere and the detection eciency of a neutron monitor. The integral resp onse function count rates is directly measured during a neutron monitor latitude survey, which is a mobile station that records counting rates while traversing a range of geomagnetic cuto s Moraal et al., 1989, and references therein. An example of count rates recorded during a typical survey is displayed in Figure 1, re- vealing a strong correlation with geomagnetic cuto . Equation 1 pro- vides a simpli ed mathematical description of the relationship b etween parameters relevant to a latitude survey in the usual approximation: 1 1 Z Z X N P ;z;t= S P; z j P; tdP = W P; z ; tdP 1: C i i T i P P C C where N P ;z;t is the neutron monitor counting rate, P is the ge- C C omagnetic cuto , z is the atmospheric depth and t represents time. issi_final6.tex; 14/01/2000; 12:50; p.2 3 S P; z represents the neutron monitor yield function for primaries i of particle typ e i and j P; t represents the primary particle rigidity i sp ectrum of typ e i at time t. It should b e emphasized that the geo- magnetic cuto P and the yield function S P; z b oth dep end on the C i arrival direction of the incident primary particles. The vertical cuto rigidity usually suces as an adequate approximation to the lower-limit rigidity of the primary sp ectrum, however there are some do cumented cases where the contribution of obliquely incident primaries are re- sp onsible for anomalies observed in latitude surveys Clem et al., 1997 and Stoker et al., 1997. The total resp onse function W P; z ; ton T the right hand side of Equation 1 is de ned as the summed pro duct of S P; z and j P; t, and has a maximum value in the range of 4- i i 7 GV at sea-level dep ending on the solar mo dulation ep o ch at time t. It should b e explicitly noted that a latitude survey can only provide a resp onse function at the time of the survey since the primary sp ectrum j P; t is time correlated with the 22 year solar cycle Fisk et al., i 1969 and Garcia-Munoz et al., 1986 and is also sub ject to sudden transient e ects suchasForbush decreases or solar energetic particles. The study of resp onse functions has lead to improved understanding of the quantities mentioned ab ove and of the neutron monitor op eration. including the additional information gained from multiplicity counts. A brief review of the neutron monitor design is discussed. 2. Background and Review of the Neutron Monitor Design In a neutron monitor, neutron sensitive prop ortional tub es, surrounded by mo derator material and a lead target, detect near-thermal neutrons pro duced lo cally from interacting incident particles. Even though neu- trons do not leave an ion trail in the prop ortional tub e, the absorption of a neutron bya nucleus is usually followed by the emission of charged particles which can b e detected. A prop ortional tub e lled with either 10 3 BF or He gas resp onds to neutrons by the exothermic reaction 3 10 7 3 3 Bn, Li or Hen,p H. The reaction cross-sections for b oth nuclei is inversely prop ortional to the neutron sp eed, having a thermal end- p oint 0.025eV of roughly 3840 barns and 5330 barns resp ectively,as shown in Figure 2. Surrounding each counter is a mo derator which serves to reduce the energy of neutrons, thus increasing the probability of an absorption in- side the counter while also providing a re ecting medium for low energy neutrons. The mo derator material is chosen to contain a signi cant frac- tion of hydrogen as the energy loss p er neutron elastic collision increases 2 4A cos dE r ecoil = with decreasing atomic mass . The neutron elastic 2 E 1+A issi_final6.tex; 14/01/2000; 12:50; p.3 4 3 10 Figure2. The reaction cross section versus neutron energy for He and B. interaction pathlength of hydrogen in typical mo derator materials is roughly 1 cm E 1 MeV and eachinteraction reduces the incident n neutron energy by a factor of 2 on the average. The lead pro ducer, which surrounds the mo derator, provides a thick large-nucleus target for inelastic interactions in which secondary neutrons are pro duced. A high atomic mass A is preferred in the pro ducer as the neutron pro duction rate p er unit mass of a material is roughly prop ortional to A with 0:7 in the 100-700 MeV incident energy range and slowly decreasing with increasing energy E 400 GeV, 0:0. Shen, 1968. Surrounding the lead is an outer mo derator, usually referred to as to the re ector, which serves to contain low energy neutrons pro duced in interactions within the lead as well as reject unwanted low energy neutrons pro duced in the lo cal surroundings from entering into the detector. In some stations each counter has a dedicated electronics interface allowing the ability to measure multiplicity coincidences b etween coun- ters which is the simultaneous o ccurrence of counts in multiple counters. The count rates of di erentmultiplicity levels can then b e recorded and analyzed Raub enheimer et al., 1980. The neutron monitor's p eak energy resp onse to secondary particles increases with increasing mul- issi_final6.tex; 14/01/2000; 12:50; p.4 5 tiplicity, suggesting eachmultiplicity level is related to the primary sp ectrum through a di erent yield function. Generally the most unstable comp onent of the neutron monitor is the Earth's atmosphere whichmust b e closely monitored at each station. The resp onse of a neutron monitor is dep endent on the air mass over-burden, and therefore meteorological changes can a ect the counting rate. Even though the neutron monitor design is optimized to detect secondary neutrons from interactions o ccurring primarily in the pro ducer, atmospheric corrections are still necessary at the few p ercent level as discussed in the next section. For further details on neutron monitor design see Stoker et al. 2000 3. Atmospheric E ects Atmospheric corrections for neutron monitors are based on theory and exp erimental investigations of meteorological phenomena that a ect the passage of particles through the atmosphere Dorman, 1970, Hat- ton, 1971 and Iucci et al., 1999.
Details
-
File Typepdf
-
Upload Time-
-
Content LanguagesEnglish
-
Upload UserAnonymous/Not logged-in
-
File Pages30 Page
-
File Size-