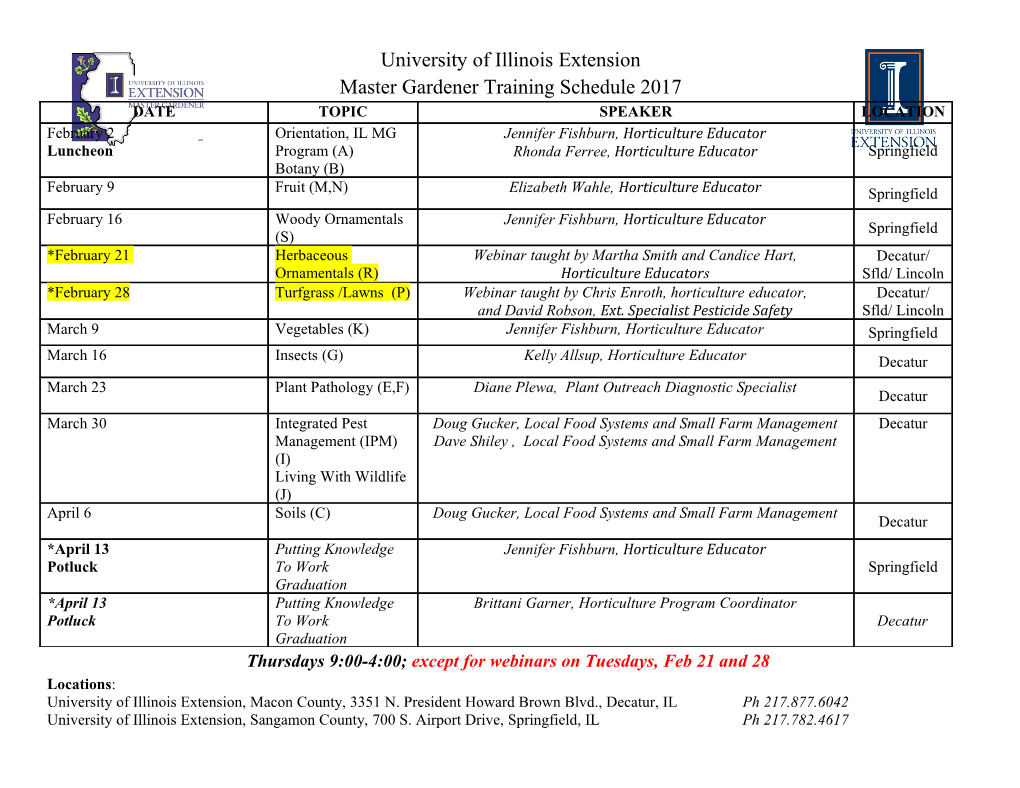
SCATTERING AND S-MATRIX 1/42 J. Rosner – Reaction Theory Summer Workshop Indiana University – June 2015 Lecture 1: Review of Lecture 2: S-matrix and Lecture 3: Some simple scattering theory related physics applications S, T , and K matrices 1-,2-channel examples Optical analogs Wave packet scattering Transmission resonances Eikonal approximation Scattering amplitude Bound states Diffractive scattering Partial wave expansion S wave properties Adding resonances Phase shifts Resonances Dalitz plot applications R-matrix Absorption Historical notes Unitarity circle Inelastic cross section Example from electronics For simplicity some of the formalism will be nonrelativistic “In” state i : Free particle in remote past | iin “Out” state j : Free particle in remote future outh | S-matrix takes “in” states to “out” (with wave packets: Goldberger and Watson, Scattering Theory, 1964.) UNITARITY; T AND K MATRICES 2/42 Sji out j i in is unitary: completeness and orthonormality of “in”≡ andh | i “out” states S†S = SS† = ½ Just an expression of probability conservation S has a piece corresponding to no scattering Can write S = ½ +2iT Notation of S. Spanier, BaBar Analysis Document #303, based on S. U. Chung et al. Ann. d. Phys. 4, 404 (1995). Unitarity of S-matrix T T † =2iT †T =2iT T †. ⇒ − 1 1 1 1 (T †)− T − =2i½ or (T − + i½)† = (T − + i½). − 1 1 1 Thus K [T − + i½]− is hermitian; T = K(½ iK)− ≡ − WAVE PACKETS 3/42 i~q ~r 3/2 Normalized plane wave states: χ~q = e · /(2π) 3 3 i(~q q~′) ~r 3 (χ , χ )=(2π)− d ~re − · = δ (~q q~ ) . q~′ ~q − ′ R Expansion of wave packet: ψ (~r, t =0)= d3q χ φ(~q ~p) ~p ~q − where φ is a weight function peaked aroundR 0 3 i~k ~r Fourier transform of φ: G(~r)= d k e · φ(~k) 3 R ψ~p(~r, t =0)= d q χ~q ~p+~p φ(~q ~p)= χ~p G(~r) . − − Norm: (ψ, ψ)=R d3q φ(~q ~p) 2 = 1 d3r G(~r) 2 =1 . | − | (2π)3 | | R R iHt 3 iEqt ψ (~r, t)= e− ψ (~r, 0) = d q φ(~q ~p)χ e− , ~p ~p − ~q 2 R 2 2 1/2 where Eq = q /2m (NR) or (q + m ) (Relativistic) . SPREADING WAVE 4/42 Expand about ~q = ~p; define ~k ~q ~p and v ∂E /∂p ≡ − i ≡ p i 2 1 ∂ Ep Eq = Ep + ~k ~v + kikj + .... · 2 ∂pi∂pj 2 iEpt 3 i~k (~r ~vt) it ∂ Ep ψ~p(~r, t)= e− χ~p d kφ(~k)e · − (1 kikj +...) − 2 ∂pi∂pj R 2 iEpt it ∂ Ep = e− χ~p(1 + i j + ...)G(~r ~vt) . 2 ∂pi∂pj ∇ ∇ − r2/2w2 Gaussian packet: G(r)= Ne− δ (r v t)(r v t) ij i− i j− j i jG(~r ~vt)= w2 + (w2)2 G(~r ~vt) . ∇ ∇ − h− i − 2 NR: ∂ Ep/(∂pi∂pj)= δij/m ; parameter describing 2 2 t ∂ Ep t L(∆k) spreading is ǫ = i jG(~r ~vt) 2 = . 2∂pi∂pj ∇ ∇ − ∼ 2mw 2p WAVE PACKET SCATTERING 5/42 E. Merzbacher, Quantum Mechanics (3rd Ed. Ch. 13) has a good discussion which will be abbreviated here 2 Free Hamiltonian: H0 = p /(2m); full: H = H0 + V 1 3 i~k (~r ~r0) Packet: ψ (~r, 0) = d k φ(~k ~k )e · − , φ(~k) ~k0 (2π)3/2 − 0 centered about 0, width ∆Rk, center of packet ~r0 At t = 0 packet is headed to target, momentum ~k0, distance ~r0 from it; want its shape for large t Expand ψ~k0(~r, 0) in eigenfunctions of H: iEnt ψ~k0(~r, 0) = n cnψn(~r) , ψ~k0(~r, t)= n cnψn(~r)e− . P P Need to find the eigenfunctions ψn(~r) GREEN’S FUNCTION 6/42 2 ~p 2 2m + V ψ = Eψ , or with k 2mE, U 2mV , ≡ ≡ Schr¨odinger equation is ( 2 + k2)ψ = Uψ ∇ ~ Define a Green’s function G(~r, r′) satisfying ( 2 + k2)G(~r, r~ )= 4πδ(~r r~ ) ∇ ′ − − ′ 1 3 ψ(~r)= d r′ G(~r, r~ )U(r~ )ψ(r~ ): particular sol’n. −4π ′ ′ ′ R i~k ~r 3/2 Add solution e · /(2π) of homogeneous equation: ~ eik·~r 1 3 ψ (~r)= d r′ G(~r, r~ )U(r~ )ψ (r~ ) ~k (2π)3/2 − 4π ′ ′ ~k ′ R Differential eqn. + boundary condx. integral equation ⇔ SPHERICAL WAVES 7/42 ~′ ~ e±ik|~r−r | Two Green’s functions: G (~r, r′)= ± ~r r~′ | − | with (+, ) (outgoing,incoming) spherical waves − ⇔ ~ Can take r′ =0; G are solutions for ~r =0 ± 6 For ~r = 0, integrate test function f(~r) times ( 2 + k2)G over small region surrounding the origin; use Gauss’∇ Law Green’s functions G define two sets of solutions: ± ~ ~′ ( ) eik·~r 1 3 e±ik|~r−r | ( ) ψ ± (~r)= d r′ U(r~ )ψ ± (r~ ) ~k (2π)3/2 − 4π ~r r~′ ′ ~k ′ R | − | where r′ is limited if U is of short range ~ 2 ~ 2 ~r r~′ Expand k ~r r′ = k r 2~r r′ + r′ kr 1 r·2 + ... | − | p − · ≃ − 1/ ~r r~ 1/r | − ′|≃ SCATTERING AMPLITUDE 8/42 ~ ( ) eik·~r 1 ikr 3 ( ) ik~′ r~′ ψ ± (~r) e± d r′ U(r~ )ψ ± (r~ )e∓ · ~k ∼ (2π)3/2 − 4πr ′ ~k ′ ~ R k′ krˆ ≡ 3 2 ( ) (2π) / 3 ( ) ik~′ r~′ Define f ± (ˆr) d r′ U(r~ )ψ ± (r~ )e∓ · ~k ≡− 4π ′ ~k ′ h i R ( ) ~ e±ikr ( ) ± 3/2 ik ~r ± Then ψ~ (~r) (2π)− e · + r f~ (ˆr) (r ) k ∼ h k i →∞ Initial flux per unit area I k 0 ∼ Final fluence I in cone of solid angle dΩ: ( ) I k(r2dΩ) f ± (ˆr)/r 2 ∼ | ~k | ( ) dσ/dΩ= I/I = f ± (ˆr) 2 differential cross section 0 | ~k | ≡ 9/42 PHASE SHIFTS δℓ ikr Large-r Schr. eq. solution: ψ eikr cos θ + f (θ)e (1) ∼ k r uℓ,k(r) m Connect with central-force solutions r Yℓ (θ,φ) where d2 ℓ(ℓ+1) 2 2 dr2 + r2 +2mV (r) k uℓ,k(r)=0 (k 2mE) h− − i ≡ Free u (r)/r R (r) solutions j (kr), n (kr) ℓ,k ≡ ℓ,k ℓ ℓ Outside range of V : Rℓ,k(r)= Aℓ jℓ(kr)+ Bℓ nℓ(kr) sin(kr ℓπ/2) cos(kr ℓπ/2) As kr R (r) A − B − →∞ ℓ,k → ℓ kr − ℓ kr sin(kr ℓπ/2+δ ) For tan δ B /A , R (r) − ℓ as kr ℓ ≡− ℓ ℓ ℓ,k ∼ kr →∞ Then ψ C (k)P (cos θ)[sin(kr ℓπ/2+ δ )]/r (2) → ℓ ℓ − ℓ ikr cosPθ ℓ Bauer: e = ℓ∞=0 i (2ℓ + 1)jℓ(kr)Pℓ(cos θ) (3) P PARTIAL WAVE EXPANSION 10/42 Compare incoming spherical wave coefficients in (2,3): ℓ iδ ψ ∞ (2ℓ + 1)i e ℓ[sin(kr ℓπ/2+ δ )]P (cos θ)/kr ≃ ℓ=0 − ℓ ℓ CompareP coeff. of outgoing spherical wave in this and (1): 2iδ (k) f (θ)= ∞ (2ℓ + 1)[(e ℓ 1)/(2ik)]P (cos θ) k ℓ=0 − ℓ P 1 iδℓ(k) = k− ℓ∞=0(2ℓ + 1)e sin δℓ(k)Pℓ(cos θ) Total cross section:P dσ 2 4π 2 σ = dΩ = dΩ f (θ) = 2 ∞ (2ℓ + 1)sin δ (k) dΩ | k | k ℓ=0 ℓ R R using dΩ Pℓ(cos θ)Pℓ′(cos θP)=4πδℓℓ′/(2ℓ + 1) R Optical theorem: σ = (4π/k)Im fk(0) Convenient to define f (k) [e2iδℓ(k) 1]/(2ik) ℓ ≡ − Then optical theorem takes the form Im f (k)= k f (k) 2 ℓ | ℓ | THE R-MATRIX 11/42 Want a real function reducing to fℓ(k) for small f Stereographic projection: R (k) (1/k)tan δ (k) ℓ ≡ ℓ 1 iδ (k) fℓ(k)= k− e ℓ sin δℓ(k) =1/k (maximum) for δℓ(k)= π [1+ikRℓ(k)]Rℓ(k) fℓ(k)= 2 2 1+k Rℓ (k) 2iδℓ(k) 1+ikRℓ(k) Sℓ(k) e = 1 ikR (k) ≡ − ℓ Many channels: real R is useful because it has eigenvalues S-matrix is useful because it is unitary: S†S =1 S = ½ +2iT = (½ + iK)/(½ iK) − UNITARITY CIRCLE 12/42 For single channel, phase shift defined by S = e2iδ. Then T = (S ½)/(2i)= − eiδ sin δ; K = tan δ = kR. The T amplitude must lie on boundary of the circle For inelastic processes 2iδ T = (ηe ½)/(2i) , η< 1. − Consider scattering in one dimension with no reflections Class of potentials giving rise to full transmission of a plane wave ψ(x) eikx incident from the left, so that as x , ψ(x) S∼(k)eikx with S(k) =1. Take 2m =1. →∞ → | | 1,2–CHANNEL EXAMPLES 13/42 2 These potls. have bound states at energies Ej = αj (1 N − ≤ j N and one can write S(k)=Πj=1[(ik αj)/(ik + ≤ 2iδ N − αj)] = e , where δ = j=1 δj and tan δj = αj/k. P Simplest one-level potential: V (x)= 2α2/cosh2α(x x ) − − 0 One-level K-matrix is just K = α/k. If we define Kj = α /k then K N K as k , but not in general. j → j=1 j →∞ Now let the potentialP permit reflections, so that ikx ikx ψ(x) Ae + Be− (x ) → ikx ikx → −∞ ψ(x) F e + Ge− (x ) → →∞ With channel 1 eikx, channel 2 e ikx, can write ∼ ∼ − F = S11A + S12G ; B = S21A + S22G TRANSMISSION RESONANCES14/42 The incoming and outgoing fluxes must be equal: F 2 + 2 2 2 | | B = A + G . This implies S†S = SS† =1. | | | | | | Square well, V (x) = V0 for x a, V (x)=0 for x > a shows transmission− resonances:| | ≤ S = S = 1 | | | 11| | 22| and S12 = S21 =0 when 2k′a = nπ (k′ = 2m(E + V0)).
Details
-
File Typepdf
-
Upload Time-
-
Content LanguagesEnglish
-
Upload UserAnonymous/Not logged-in
-
File Pages44 Page
-
File Size-