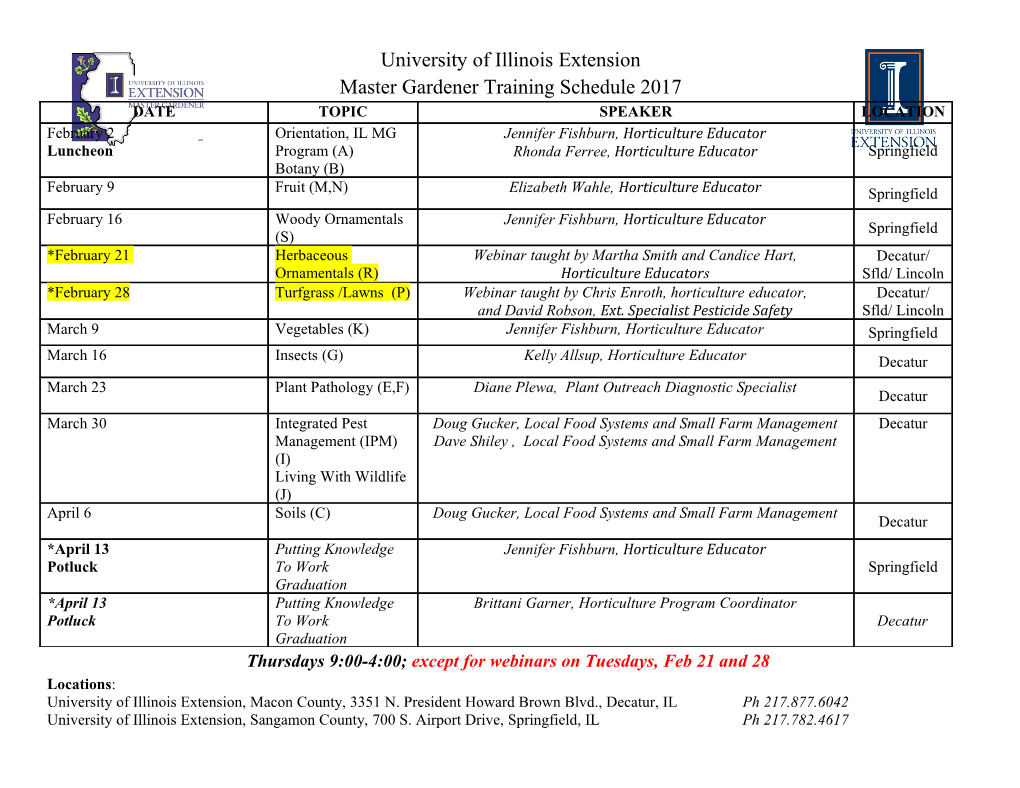
74 XPS STUDIES OF VALENCE STATES OF LANTHANIDE AND ACTINIDE ELEMENTS IN SYNROC C J. Szajman, S. Myhra and R.St.C. Smart School of Science, Griffith University Nathan Q. 4111 ABSTRACT High level nuclear waste (HLW) is simulated in part by addition of small quantities of lanthanides and actinides to the Synroc precursor slurry. There are two main parameters which influence the crystallographic destination of the HLW species - the cation size and its valence state. X-ray photoelectron spectro- scopy (XPS) was used to investigate the valence states of rare earth elements (REE) in Synroc. Experimental difficulties are considerable at concentrations approaching detection limits in a solid with up to 20 different elements. Some successful observations will be described and their implications for the struct- ural chemistry will be discussed. INTRODUCTION Ringwood et al has developed a titanate ceramic (SYNROC) material which will immobilize high level nuclear waste (HLW) by trapping the radionuclides in a matrix of composite minerals. As part of the description of SYNROC, we have studied the valence states of some HLW elements in order to infer their elemental destinations in the mineral crystal structure. X-ray photoelectron spectroscopy (XPS) has now become a widely used tech- nique for studying electronic structure. The spectrum (electron intensity versus energy) has a great wealth of information about solids: chemical state, atomic concentrations, identification of constituent elements, binding energy of elect- ron orbitals, density of states are just a few applications. RESULTS AND DISCUSSION The peak position (binding energy) in the XPS spectrum indicates the valence state of the atom. The binding energy normally increases with higher valence states as the electrons are then more tightly bound due to the presence of the additional nuclear charge. SYNROC C (No. 193) contains 15 simulated waste elements and a number of processing contaminants such as sodium, lead and carbon. If the matrix minerals are included, there are well over 20 elements present. Identification of valence states in SYNROC is sometimes not possible as, for example, phosphorus where the signal is totally obscured by the stronger strontium signal. An additional complication is the difficulty of detection of very low atomic concentrations as is the case for HLW elements. A spectrum of the Ce 3d level in SYNROC C was acquired over a long time (12 hr) and shows a complicated structure of more than six primary and satellite peaks. A background spectrum from SYNROC B (containing the same matrix elements as SYNROC C but with no added waste) was subtracted and the resultant spectrum is shown in Fig. l(a). 75 Cerium and some other lanthanides show strong satellite peaks due to inter- action of the 3f electrons with the ligand atoms. Particularly interesting is the 3d spectrum of Ce (as CeO2) shown in Pig. l(b) (all peaks are charge shift ed by 4.9 eV) . The peak located at 920. 4eV is always present in the spectrum of Ce'l+ and never in Ce +, while the metallic form of ceriun. is thermodynamically very unlikely and would appear at lower binding energy. This allows unambiguous identification of the valence state of Ce. In SYNROC C, the lack of the high energy peak and the binding energies for 3d peaks show unequivocally that Ce is 3 incorporated in the lower, trivalent state. Spectra obtained by Barr from Ce203 have very similar binding energies and identical shape to the SYNROC case as shown in Fig. l(c). On the other hand, the valence state of uranium is harder to determine as there are no characteristic satellites associated with a particular valence state. The uranium spectrum in SYNROC is shown in Fig. 2. The binding of U 4fy/2' corrected for slight charging, is 379.7 e.v. This is in excellent agree ment with 379 . 6 eV for U^+ , determined by Verbist et al'* and 379 . 5 eV measured in this laboratory. If, in fact, a hexavalent species were present then we might expect higher binding energy at 381eV. Together, the Ca and Zr sites in zirconolite are capable of incorporating the trivalent lanthanide and actinide fission products , but the occupancy will depend on their ionic radius. Since Ca2+ has a larger radius (1.26 &) than Zr (0.92 A) it is likely that lighter, smaller lanthanides will substitute for Ca2+ + Zr4+ -> large REE3+ + Ti3+ Ca2+ + Zr4"1' -»• 2 x small REE3+. An alternative site for these elements is perovskite, which can immobilize divalent, trivalent and tetravalent ions. Here Ce3+ will go into Ca2+ sites with Ti changing to Ti3+ to maintain the charge balance. However, a tetravalent ion like U1* can directly substitute for Zr in zirconolite. In perovskite, the substitution of tetravalent Ti is straighf orward , in theory, although a little hexavalent U may be present to balance the charge. The reducing conditions during calcination favour lower valence. If U6+ is indeed present, then its concentration is less than 1/20 of U +. CONCLUSION . The valence of Ce and U in SYNROC C has been determined using XPS. Since cerium in SYNROC does not have the characteristic Ce4+ satellite it is in the trivalent state. Careful binding energy measurements show that uranium in SYNROC is tetravalent. Therefore, Ce and U waste species are likely to be incorporated in both the zirconolite and perovskite phases of SYNROC. 76 REFERENCES 1. A.E. Ringwood, V.M. Oversby, S.E. Kesson, W. Sinclair, N. Ware, W. Hiberson, and A. Major. Nucl. Chem. Waste Manage., 2, (1981) 287-305. 2. P. Burroughs, A. Harnmett, A.F. Orchard, G. Thornton, Journal of Chemical Society, Dalton (1976) 1686. 3. T.L. Barr, Quantitative Surface Analysis of Materials. ASTM STP 643., N.S. Mclntyre, Ed., American Society for Testing and Materials (1978), 83-104. 4. J. Verbist, J. Riga, J.J. Preaux and R. Caudano, J. Electron Spectrosc. and Rel. Phenom. 5^ (1974), 193. 5. T.S. White, P. Fielding to be published. US 110 IIS 110 MS 900 B9S 890 US 880 87S 910 III 9t 1)1 191 190 Ml m 161 141 180 178 171 FIGURE CAPTIONS Fig. 1 XPS spectra of cerium in: (a) SYNROC C. Note that there is no charac- teristic satellite peak at 916 eV; (b) CeO2, Ce is in the tetravalent state showing a strong satellite peak at 920.4 eV (charging causes 4.9 eV shift in the binding energy); Ce203 (ref. 4). Spectrum's features ajre identical to Fig. l(a). Fig. 2 Spectrum of uranium 4f level in SYNROC C. There is 0.5 eV charging shift towards the higher binding energy..
Details
-
File Typepdf
-
Upload Time-
-
Content LanguagesEnglish
-
Upload UserAnonymous/Not logged-in
-
File Pages3 Page
-
File Size-