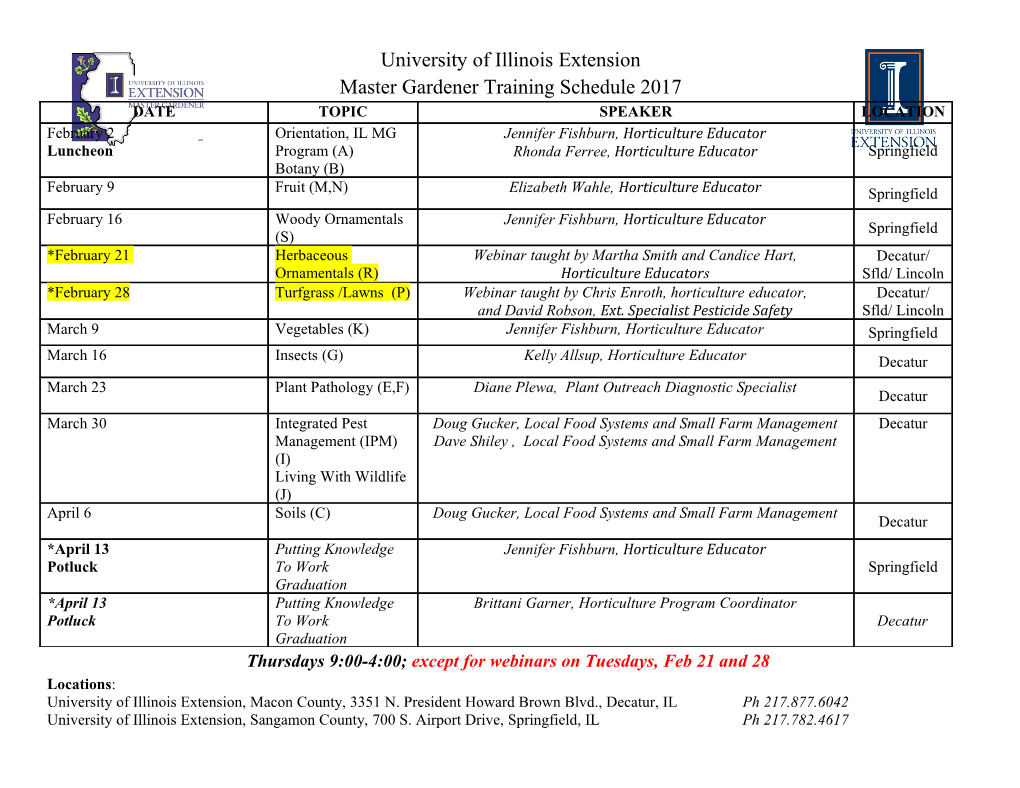
CHAPTER 8. ENZYMES: BASIC CONCEPTS AND KINETICS coelenterazine The activity of an enzyme is responsible for the glow of the luminescent jellyfish at left. The enzyme aequorin catalyzes the oxidation of a compound by oxygen in the presence of calcium to release CO2 and light CHAPTER 8. ENZYMES: BASIC CONCEPTS AND KINETICS Introduction . Enzymes are large biological molecules that catalyze chemical transformations . The molecule also mediates the transformation of one form of energy into another . 25% of the genes in the human genome encode enzymes . Catalytic power and specificity – important characteristics . Active site – the place where enzymatic reactions take place CHAPTER 8. ENZYMES: BASIC CONCEPTS AND KINETICS Introduction . Nearly all known enzymes are proteins • RNA can function as a catalyst . Proximity effect – enzymes bring substrates closer together in an optimal orientation . They catalyze reactions by stabilizing transition states 8.1 CATALYTIC POWER AND SPECIFICITY Rate enhancement by enzymes . Enzymes accelerate reactions by factors of as much as a million or more 8.1 CATALYTIC POWER AND SPECIFICITY Substrate specificity . A substrate is a molecule upon which an enzyme acts . Some proteases cleaves specific amide bonds in a peptide . The specificity of an enzyme is due to the precise interaction of the substrate with the enzyme . This precision is a result of the intricate 3D structure of the enzyme protein Fig 8.1 Enzyme specificity. (A) Trypsin cleaves on the carboxyl side of arginine and lysine residues, whereas (B) thrombin cleaves Arg-Gly bonds in particular sequences only 8.1 CATALYTIC POWER AND SPECIFICITY Cofactors . The catalytic activity of enzymes depends on the presence of small molecules termed cofactors . Two groups of cofactors: metals and small organic molecules (coenzymes) . Tightly bound coenzymes are called prosthetic groups 8.1 CATALYTIC POWER AND SPECIFICITY Energy transformation . Enzymes can transform energy from one form into another . Photosynthesis – conversion of light E into chemical-bond E . Cellular respiration – conversion of the E in food into an ion gradient and the E in ATP . Myosin converts the E of ATP into the mechanical E of contracting muscles . Pumps in the membranes use the E of ATP to transport molecules and ions across the membrane . Enzymes play fundamental roles in these processes 8.2 FREE ENERGY Free energy change . The free energy change (ΔG) of a reaction tells us if the reaction can take place spontaneously • A reaction can take place spontaneously only if ΔG<0 • A system is at equilibrium if ΔG=0 • A reaction cannot take place spontaneously if ΔG>0 • ΔG depends only on the free energy of the products minus the free energy of the reactants; independent of the path of the transformation • The ΔG provides no information about the rate of a reaction • The reaction rate depends on the free energy of activation (ΔG‡) 8.2 FREE ENERGY Free energy and equilibrium . Consider a reaction A + B ← → C + D [C][D] G = G + RT ln G: standard free energy change [A][B] . If we define G' as the standard free energy change at pH=7 [C][D] G = G' + RT ln [A][B] . At equilibrium, [C][D] G' = −RT ln = −RT lnK' [A][B] eq −G'/RT K'eq = e 8.2 FREE ENERGY Free energy and reaction rate . Enzymes alter only the reaction rate and not the reaction equilibrium . Enzymes accelerate the attainment of equilibria but do not shift their positions . The equilibrium position is a function only of the ΔG between reactants and products Fig 8.2 Enzymes accelerate the reaction rate. The same equilibrium point is reached but much more quickly in the presence of an enzyme. 8.3 THE TRANSITION STATE Enzyme and activation energy . A chemical reaction of substrate S to form product P goes through a transition state, X‡ S → X‡ → P . The difference in free energy between the transition state and the substrate is called the activation energy, ΔG‡ ‡ ‡ ΔG = GX − GS Fig 8.3 Enzymes decrease the activation energy. Enzymes accelerate reactions by decreasing ΔG‡, the free energy of activation. 8.3 THE TRANSITION STATE Enzyme-substrate complex . Much of the catalytic power of enzymes comes from their bringing substrates together in favorable orientations . The enzyme-substrate complex can be seen by the following results: 1. The fact that an enzyme- catalyzed reaction has a maximal velocity suggests the formation of a discrete ES complex Fig 8.4 Reaction velocity vs substrate concentration in an enzyme-catalyzed reaction. 8.3 THE TRANSITION STATE Enzyme-substrate complex . The enzyme-substrate complex can be seen by the following results: 2. X-ray crystal structure of an enzyme-substrate complex 3. Spectroscopic studies Fig 8.5 Structure of cytochrome P450 complexed with its substrate camphor. 8.3 THE TRANSITION STATE The active sites of enzymes . The active site of an enzyme is the region that binds the substrates . The interaction of the enzyme and substrate at the active site promotes the formation of the transition state and lowers the ΔG‡ of the reaction . There are some common features in the active sites of enzymes 1. The active site is a three dimensional cleft formed by groups that come from different parts of the amino acid sequence Fig 8.6 Active sites may include distant residues. 8.3 THE TRANSITION STATE The active sites of enzymes . There are some common features in the active sites of enzymes 2. The active site takes up a small part of the total volume of an enzyme – the rest parts serve as a scaffold and regulatory sites 3. Active sites are unique microenvironments – optimized for substrate binding and catalysis 4. Substrates are bound to enzymes by multiple weak attractions – electrostatic, H bonds, and van der Waals forces 8.3 THE TRANSITION STATE The active sites of enzymes . There are some common features in the active sites of enzymes 5. The specificity of binding depends on the precisely defined arrangement of atoms in an active site Fig 8.8 Lock-and-key model of enzyme- Fig 8.9 Induced-fit model of enzyme- substrate binding. Proposed by Emil Fischer substrate binding. Proposed by Daniel in 1890. Koshland in 1958. 8.3 THE TRANSITION STATE Substrate binding energy . Free energy is released by the formation of a large number of weak interactions between a complementary enzyme and its substrate . The full complement of such interactions is formed only when the substrate is converted into the transition state . The maximal binding energy is released when the enzyme facilitates the formation of the transition state – lowering activation energy 8.4 THE MICHAELIS-MENTEN MODEL First-order reactions . A first-order reaction is one whose rate depends on the concentration of a single reactant raised to the first power . Consider a reaction: A → P . We can express the rate law V = k[A] 8.4 THE MICHAELIS-MENTEN MODEL Second-order reactions . A second-order reaction is one whose rate depends on the concentration of two different reactants, each raised to the first power . Consider a reaction: 2A → P or A + B → P . We can express the rate law V = k[A]2 or V = k[A][B] . pseudo-first order reactions ([A] << [B]) – no dependence of the rate on [B] 8.4 THE MICHAELIS-MENTEN MODEL Initial velocity and substrate concentration . The simplest way to investigate the reaction rate is to follow the increase in reaction product as a function of time . Initial velocity (V0) is measured in different concentration ([S]) of the substrate and V0 is plotted as a function of [S]; typically saturation curve is observed in high [S] Fig 8.10 Determining the relation between initial velocity and substrate concentration. 8.4 THE MICHAELIS-MENTEN MODEL The Michaelis-Menten equation . Conditions • The concentration of the enzyme is negligible • The initial rate of formation is measured Fig 8.11 Michaelis-Menten kinetics. 8.4 THE MICHAELIS-MENTEN MODEL Steady State Kinetics . The steady state is the situation in which the value of a particular quantity is constant • Its rate of formation is balanced by its rate of destruction • The population of a country in a steady state • In enzyme kinetics, the concept is applied to the concentration of enzyme-bound intermediates Pre-steady state Once the intermediates reach their steady state concentrations, the reaction rate changes relatively slowly with time. During this steady state, the rates of enzymatic reactions are traditionally measured 8.4 THE MICHAELIS-MENTEN MODEL The Michaelis-Menten equation Rate of formation of ES Rate of breakdown of ES If we use the steady state approximation for [ES] Rate of formation of ES = Rate of breakdown of ES The equation is rearranged and the ratio of the rate constants is ····· 1 defined as KM. 8.4 THE MICHAELIS-MENTEN MODEL The Michaelis-Menten equation Because we measure initial velocities, [S] is equal to the initial [S]. Because we cannot measure [E], [E] is needed to be expressed by measurable quantities. (Enzyme exists in the two forms) ····· 2 By using Eq. 1 and 2, [ES] is expressed as: 8.4 THE MICHAELIS-MENTEN MODEL The Michaelis-Menten equation V0 becomes the maximum when all enzymes are bound to S. ([ES] = [E]0) . Note th 1. When [S]>>KM, V0 = Vmax (0 order) When [S]<<KM, V0 = Vmax [S]/KM (1st order for [S]) 2. When [S] = KM, V0 = Vmax/2 Therefore, KM is equal to the substrate concentration at which the reaction rate is half its maximal value 8.4 THE MICHAELIS-MENTEN MODEL KM of aldehyde dehydrogenase . Ethanol-sensitive persons exhibit facial flushing and rapid heart rate (tachycardia) after ingesting alcohol . In the liver, alcohol dehydrogenase converts ethanol into acetaldehyde . The acetaldehyde is the cause of the symptoms and processed to acetate by aldehyde dehydrogenase .
Details
-
File Typepdf
-
Upload Time-
-
Content LanguagesEnglish
-
Upload UserAnonymous/Not logged-in
-
File Pages65 Page
-
File Size-