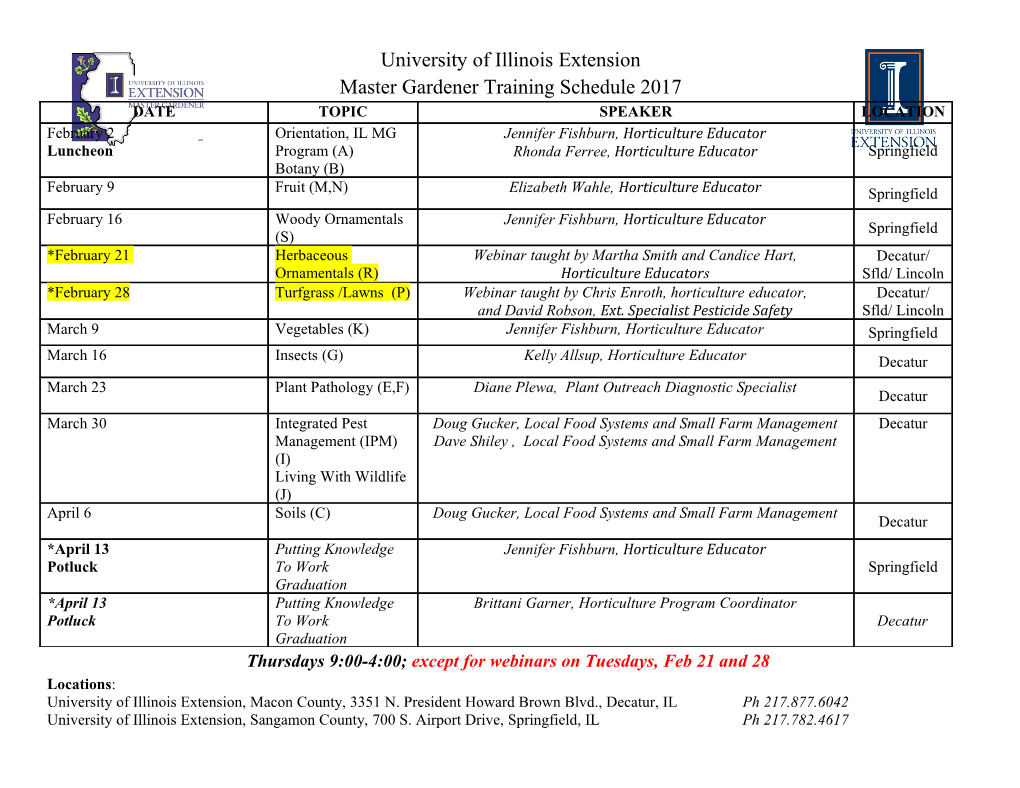
MASSACHUSETTS INSTITUTE OF TECHNOLOGY Department of Physics String Theory (8.821) – Prof. J. McGreevy – Fall 2007 Solution Set 4 String scattering, open strings, supersymmetry warmup. Reading: Polchinski, Chapter 6. Please see course webpage for supersymmetry refs. Due: Thursday, October 25, 2007 at 11:00 AM, in lecture or in the box. 1. Another tree amplitude (Polchinski 6.11). µ (a) For what values of ζµ,ν and k does the vertex operator (k)= ccg˜ ′ ζ (k) : ∂Xµ∂X¯ ν eik·X : Oζ c µν create a physical state of the bosonic string ? We showed in class that an operator of the above form creates a physical state as long as the object multiplying cc˜ (let’s call it = µ ν ik·X V ζµν(k) : ∂X ∂X¯ e :) is a conformal primary operator of weight (1,1). Let’s check: α′k2/4+1 1 1 T (z) (0) = (0) + ∂ (0) + ζ α′kµ : ∂X¯ ν eik·X : zz V z2 V z V z2 µν The extra term on the RHS vanishes if µ ζµνk =0, and in that case is a primary of weight 1+ α′k2/4 . Acting with T , V z¯z¯ we require ν ζµνk =0. If this condition isn’t satisfied, is in fact a descendant: V µ ν ik·X ν ik·X ikµ : ∂X ∂X¯ e : = L−1 : ∂X¯ e : Note that this is also the condition that the polarization isn’t lon- gitudinal, i.e. gauge trivial in the target space: target space gauge 1 symmetry is the first of the many conditions from the Vir constrat- ints. Also we require k2 =0, so h =1. (b) Compute the three-point scattering amplitude for a massless closed string and two closed-string tachyons at tree level. 2 ′ −2λ ik2·X ik3·X 2 (k ,ζ; k ,k )= g g e ζ (k; z , z¯ ):˜cce (z , z¯ )::cce ˜ (z , z¯ ) : 2 . AS 1 2 3 c c µν Oζ 1 1 2 2 3 3 S 3 3 gh 2 gh c(z )˜c(z ) 2 = z C 2 . h i i iS | ij| S i=1 i<j Y Y ¯ ik1·X ik2·X ik3·X X : ∂X∂Xe (z1, z¯1) :: e (z2, z¯2) :: e (z3, z¯3) : S2 = 3 3 ′ µ µ ′ µ µ ′ X α ki·kj iα k2 k3 iα k2 k3 iC 2 δ˜( k ) z + + S i | ij| · − 2 z z − 2 z¯ z¯ i<j 12 13 12 13 X Y Now we play with the kinematics. The mass-shell conditions are ′ 2 ′ 2 ′ 2 α k1 =0, α k2 = α k3 = +4. These imply that k k = k k =0, α′k k =4. 1 · 2 1 · 3 2 · 3 gh 2 X −2λ 8π Using this and the relation CS = CS2 CS2 e = α′g2 , and the condition µ c derived in part (a), ζµνk1 =0, the 3-point amplitude can therefore be written as z z 2 kµ kµ kµ kµ = i2πα′g′ δ˜( k)ζ 12 13 2 + 3 2 + 3 A − c µν z z z z¯ z¯ 23 12 13 12 13 X πiα′ = g′ δ˜ 26 k ζ kµ kν . − 2 c µν 23 23 X (c) Factorize the tachyon four-point amplitude on the massless pole (in say ′ the s-channel), and use unitarity to relate the massless coupling gc to gc, the coupling for the tachyon. The Virasoro amplitude is 2 4 ′ ′ (4) 8πig α u α t c ˜26 2 − 2 −2 − 2 −2 S2 (k1, ..k4)= δ ( k) d z4 z4 1 z4 A α′ C | | | − | X Z 2 The massless s-channel pole comes from the next-to-leading term in the expansion of the integrand near z4 ; alternatively, we can see it from properties of gamma functions→ as ∞ follows. ′ ′ ′ 2 4 α s α t α u (4) 8πigc 26 Γ 1 4 Γ 1 4 Γ 1 4 2 = δ˜ ( k)2π − − ′ − − ′ − − ′ AS α′ Γ 2 α s Γ 2 α t Γ 2 α u 4 4 4 X − − − − − − 1 Near x = 1, Γ(x) x+1 , so the massless s-channel pole (where α′(t + u) −16 ) comes∼ − from ∼ − ′ α s s∼0 4 Γ 1 . − − 4 ≃ α′s Using Γ(x +1) = xΓ(x), the rest of the gammas give: ′ ′ α t α u ′ ′ ′ 2 1 Γ 1 4 Γ 1 4 α t α u α − − ′ − − ′ = 2 2 = 4+ tu. Γ(2) Γ 2 α t Γ 2 α u − − 4 − − 4 − 2 − − 4 − − 4 16 2 We will later realize that we should rewrite this using ′ =(u+t) = − α (u t)2 +4ut as − α′ 2 α′ 2 4+ tu = (u t)2. − 2 − 4 − So the four point factorizes as ′ 2 2 2 4 α (u t)2 . (4) ′ 16π igc ˜26 4 (α s 0) ′ δ ( k) − ′ − . (Elmo) A ∼ ∼ α ( α s/4) ! X − The optical theorem says 26 d k S2 (k1,k2; k,ζ) S2( k,ζ; k3,k4) 2 (k ..k )= i A A − + reg. at s =0. AS 1 4 (2π)26 k2 + iǫ ζ Z X − Using the above expression for (3), this is A 2 ′ 2 µ ν ⋆ ρ λ π (α ) ζµνk k ζ k k = i (g′ )2δ˜( k) 12 12 ρλ 34 34 Aunitarity − 4 c s + iǫ ζ X X 3 µν The sum over polarizations ζ runs over normalized ζµνζ = 1 two- tensors which are transverse to the momentum of the intermediate state k = k1 + k2: µ ν ζµνk =0= ζµνk . One basis element for the space of such tensors is k12µk12ν eµν = 2 k12 2 2 which is transverse because k1 = k2. The sum over polarizations is S ζ kµ kν ζ⋆ kρ kλ ≡ µν 12 12 ρλ 34 34 ζ X In fact none of the other basis elements contribute, and we get S =(k k )2 . 12 · 34 Alternatively, just as the sum over polarizations of photons (see e.g. Srednicki p.358) can be replaced with ǫ (k)ǫ⋆(k) η µ ν → µν ǫ X when appearing in gauge-invariant amplitudes (the terms that are missing on the LHS don’t couple by gauge invariance), here we would find similar rule of the form ζ ζ⋆ η η + η η , µν ρσ → µρ νσ µσ νρ ζ X which reproduces the result above. Some kinematical voodoo gives S =(k k )2 =(k k k k + k k k k )2 =(u t)2. 12 · 34 1 · 3 − 2 · 3 2 · 4 − 1 · 4 − This leaves us with π2(α′)2 (u t)2 = i (g′ )2δ˜( k) − (Kermit) Aunitarity − 4 c s + iǫ X 4 Comparing (Elmo) and (Kermit) leads to the relation 2 g′ = g , c α′ c ′ which lets us eliminate gc. Such a relation exists for every closed string state. 2. High-energy scattering in string theory.1 Consider the tree-level scattering amplitude of N bosonic string states, with µ momenta ki : n n (n)( k , ..., k )= d2z (z , z¯ ) . A { 1 n} i Vki i i i=4 *i=1 + 2 Z Y Y S In this problem we will study the limit of hard scattering (also called fixed-angle scattering), where we scale up all the momenta uniformly, kµ αkµ, α ; i 7→ i →∞ in terms of the Lorentz-invariant Mandelstam variables, we are taking the limit sij ,sij/skl fixed. It was claimed in class that the 4-point function behaves ′ in this→∞ limit like e−sα f(θ) where θ is the scattering angle. In this limit, k2 m2 ≫ (if both aren’t zero) and we can ignore the parts of the vertex operators other than eik·X . (a) Notice that the integral over X is always gaussian, hence the action of the saddle point solution gives the exact answer. Find the saddle-point configura- tion of X(z) and evaluate the on-shell action. n n (n)( k , ..., k )= d2z : eiki·X (z , z¯ ) : . A { 1 n} i i i i=4 *i=1 + 2 Z Y Y S n 1 2 ¯ iki·X − 2 ′ R d z(∂X·∂X+J·X) : e (zi, z¯i) : = [DX] e πα *i=1 + 2 Y S Z with J µ = 2πα′i kµδ2(z z ). − i − i i X 1This discussion follows the papers of Gross and Mende. 5 Complete the square: 1 ∂X∂X¯ + J X = ∂X˜∂¯X˜ J 2 · − 4 with 1 X˜(z) X(z)+ d2z′G(z, z′)J(z′) ≡ 2 Z where G is the Green function: ∂∂G¯ = δ2. Under this linear field redefinition, the measure is [DX˜] = [DX] and we find n 2 1 2 J iki·X − ′ R d z 4 : e (zi, z¯i) : = e 2πα Z (Fred) *i=1 + 2 Y S where 1 2 − ′ R d z ∂X˜·∂¯X˜ Z = [DX˜] e 2πα ( ) Z is just a number; the saddle point for X˜ is at X˜ = 0, which means the saddle for X is at 1 X = d2z′G(z, z′)J(z′). ⋆ −2 Z The Green function on the sphere is 1 G(z, z′)= ln z z′ 2 −2π | − | so plugging in our expression above for J we get n 1 α′ Xµ = d2z′ ln z z′ 2J(z′)µ = i kµ ln z z 2. ⋆ 4π | − | − 2 i | − i| i=1 Z X Notice that it’s imaginary! Crazy! Nevertheless we’ll see that it gives the correct answer. Another expression which is sometimes useful is n 1 Xµ(z)= d2z′G(z, z′)J(z′)µ = πiα′ G(z, z )kµ (Eldridge).
Details
-
File Typepdf
-
Upload Time-
-
Content LanguagesEnglish
-
Upload UserAnonymous/Not logged-in
-
File Pages21 Page
-
File Size-