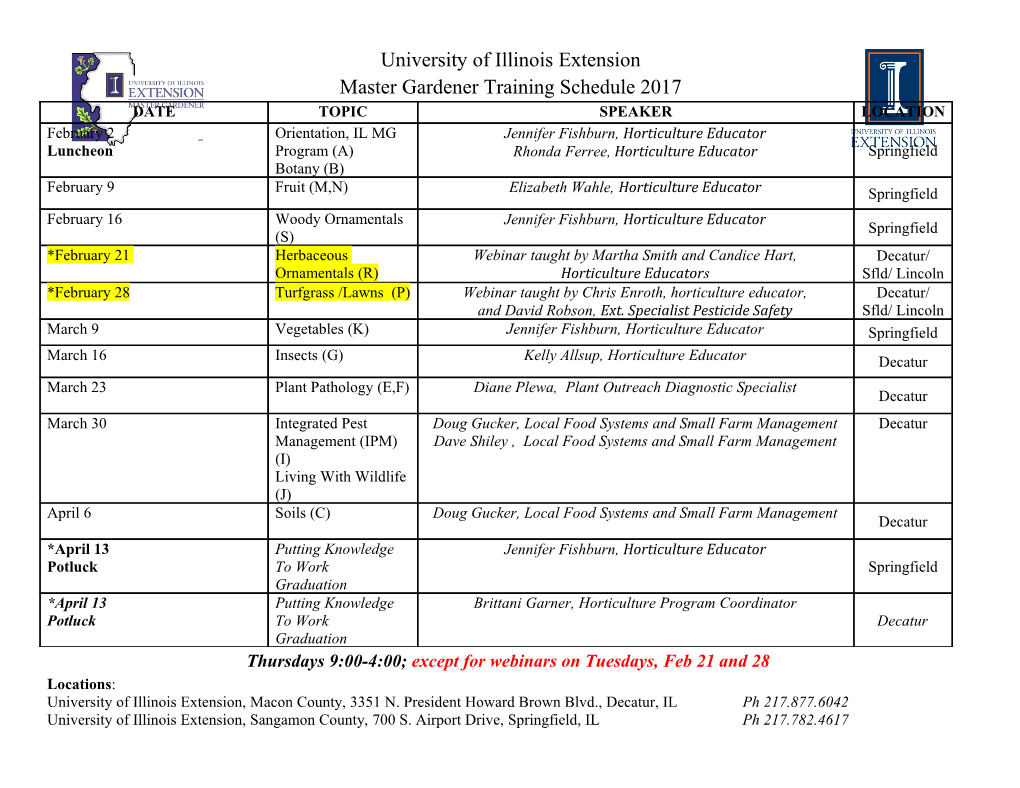
CHAPTER 4 RADIONUCLIDE PRODUCTION H.O. LUNDQVIST Department of Radiology, Oncology and Radiation Science, Uppsala University, Uppsala, Sweden 4.1. THE ORIGINS OF DIFFERENT NUCLEI All matter in the universe has its origin in an event called the ‘big bang’, a cosmic explosion releasing an enormous amount of energy about 14 billion years ago. Scientists believe that particles such as protons and neutrons, which form the building blocks of nuclei, were condensed as free particles during the first seconds. With the decreasing temperature of the expanding universe, the formation of particle combinations such as deuterium (heavy hydrogen) and helium occurred. For several hundred million years, the universe was plasma composed of hydrogen, deuterium, helium ions and free electrons. As the temperature continued to decrease, the electrons were able to attach to ions, forming neutral atoms and converting the plasma into a large cloud of hydrogen and helium gas. Locally, this neutral gas slowly condensed under the force of gravity to form the first stars. As the temperature and the density in the stars increased, the probability of nuclear fusion resulting in the production of heavier elements increased, culminating in all of the elements in the periodic table that we know today. As the stars aged, consuming their hydrogen fuel, they eventually exploded, spreading their contents of heavy materials around the universe. Owing to gravity, other stars formed with planets around them, composed of these heavy elements. Four and a half billion years have passed since the planet Earth was formed. In that time, most of the atomic nuclei consisting of unstable proton–neutron combinations have undergone transformation (radioactive decay) to more stable (non-radioactive) combinations. However, some with very long half-lives remain: 40K, 204Pb, 232Th and the naturally occurring isotopes of uranium. The discovery of these radioactive atoms was first made by Henri Becquerel in 1896. The chemical purification and elucidation of some of the properties of radioactive substances was further investigated by Marie Skłodowska-Curie and her husband Pierre Curie. Since some of these long lived radionuclides generated more short lived radionuclides, a new scientific tool had been 117 CHAPTER 4 discovered that was later found to have profound implications in what today is known as nuclear medicine. George de Hevesy was a pioneer in demonstrating the practical uses of the new radioactive elements. He and his colleagues used a radioactive isotope of lead, 210Pb, as a tracer (or indicator) when they studied the solubility of sparingly soluble lead salts. De Hevesy was also the first to apply the radioactive tracer technique in biology when he investigated lead uptake in plants (1923) using 212Pb. Only one year later, Blumengarten and Weiss carried out the first clinical study, when they injected 212Bi into one arm of a patient and measured the arrival time in the other arm. From this study, they concluded that the arrival time was prolonged in patients with heart disease. 4.1.1. Induced radioactivity In the beginning, nature was the supplier of the radioactive nuclides used. Isotopes of uranium and thorium generated a variety of radioactive heavy elements such as lead, but radioactive isotopes of light elements were not known. Marie Curie’s daughter Irène, together with her husband Frédéric Joliot took the next step. Alpha emitting sources had long been used to bombard different elements, for example, by Ernest Rutherford who studied the deflection of α particles in gold foils. The large deflections observed in this work led to the conclusion that the atom consisted of a tiny nucleus of protons with orbiting electrons (similar to planets around the sun). However, Joliot–Curie also showed that the α particles induced radioactivity in the bombarded foil (in their case, aluminium foil). The induced radioactivity had a half-life of about 3 min. They identified the emitted radiation to be from 30P created in the nuclear reaction 27Al(α, n)30P. They also concluded that: “These elements and similar ones may possibly be formed in different nuclear reactions with other bombarding particles: protons, deuterons and neutrons. For example, 13N could perhaps be formed by capture of a deuteron in 12C, followed by the emission of a neutron.” The same year, this was proved to be true by Ernest Lawrence in Berkeley, California and Enrico Fermi in Rome. Lawrence had built a cyclotron capable of accelerating deuterons up to about 3 MeV. He soon reported the production of 13N with a half-life of 10 min. Thereafter, the cyclotron was used to produce several other biologically important radionuclides such as 11C, 32P and 22Na. Fermi realized that the neutron was advantageous for radionuclide production. Since it has no charge, it could easily enter into the nucleus and induce a nuclear reaction. He immediately made a strong neutron source by sealing up 232Ra gas 118 RADIONUCLIDE PRODUCTION with beryllium powder in a glass vial. The α particle emitted from 232Ra caused a nuclear reaction in beryllium and a neutron was emitted, 9Be(α, n)12C. Fermi and his research group started a systematic search by irradiating all available elements in the periodic system with fast and slow neutrons to study the creation of induced radioactivity. From hydrogen to oxygen, no radioactivity was observed in their targets, but in the ninth element, fluorine, their hopes were fulfilled. In the following weeks, they bombarded some 60 elements and found induced radioactivity in 40 of them. They also observed that the lighter elements were usually transmuted into radionuclides of a different chemical element, whereas heavier elements appeared to yield radioisotopes of the same element as the target. These new discoveries excited the scientific community. From having been a rather limited technique, the radioactivity tracer principle could suddenly be applied in a variety of fields, especially in life sciences. De Hevesy immediately started to study the uptake and elimination of 32P phosphate in various tissues of rats and demonstrated, for the first time, the kinetics of vital elements in living creatures. Iodine-128 was soon after applied in the diagnosis of thyroid disease. This was the start of the radiotracer technology in biology and medicine as we know it today. One early cyclotron produced nuclide of special importance was 11C since carbon is fundamental in life sciences. Carbon-11 had a half-life of only 20 min but by setting up a chemical laboratory close to the cyclotron, organic compounds labelled with 11C were obtained in large amounts. Photosynthesis 11 was studied using CO2 and the fixation of carbon monoxide in humans by inhaling 11CO. However, 20 min was a short half-life and the use of 11C was limited to the most rapid biochemical reactions. It must be remembered that the radio-detectors used at that time were primitive and that the chemical, synthetic and analytical tools were not adapted to such short times. A search to find a more long lived isotope of carbon resulted in the discovery in 1939 of 14C produced in the nuclear reaction 13C(d, p)14C. Unfortunately, 14C produced this way was of limited use since the radionuclide could not be separated from the target. However, during the bombardments, a bottle of ammonium nitrate solution had been standing close to the target. By pure chance, it was discovered that this bottle also contained 14C, which had been produced in the reaction 14N(n, p)14C. The deuterons used in the bombardment consist of one proton and one neutron with a binding energy of about 2 MeV. When high energy deuterons hit a target, it is likely that the binding between the particles breaks and that a free neutron is created in what is called a ‘stripping reaction’. The bottle with ammonium nitrate had, thus, unintentionally been neutron irradiated. Since no carbon was present in the bottle (except small amounts from solved airborne 119 CHAPTER 4 carbon dioxide), the 14C produced this way was of high specific radioactivity. It was also very easy to separate from the target. In the nuclear reaction, a 14 ‘hot’ carbon atom was created, which formed CO2 in the solution. By simply bubbling air through the bottle, the 14C was released from the target. The same year, tritium was discovered by deuteron irradiation of water. One of the pioneers ‘Martin Kamen’ stated: “Within a few months, after the scientific world had somewhat ruefully concluded that development of tracer techniques would be seriously handicapped because useful radioactive tracers for carbon, hydrogen, oxygen and nitrogen did not exist, 14C and 3H were discovered”. Before the second world war, the cyclotron was the main producer of radionuclides since the neutron sources at that time were very weak. However, with the development of the nuclear reactor, that situation changed. Suddenly, a strong neutron source was available, which could easily produce almost unlimited amounts of radioactive nuclides including biologically important elements, such as 3H, 14C, 32P and 35S, and clinically interesting radionuclides, such as 60Co (for external radiotherapy) and 131I, for nuclear medicine. After the war, a new industry was born which could deliver a variety of radiolabelled compounds for research and clinical use at a reasonable price. However, accelerator produced nuclides have a special character, which makes them differ from reactor produced nuclides. Today, their popularity is increasing again. Generally, reactor produced radionuclides are most suitable for laboratory work, whereas accelerator produced radionuclides are more useful clinically. Some of the most used radionuclides in nuclear medicine, such as 111In, 123I and 201Tl, and the short lived radionuclides, 11C, 13N, 15O and 18F, used for positron emission tomography (PET), are all cyclotron produced. 4.1.2. Nuclide chart and line of nuclear stability During the late 19th century, chemists learned to organize chemical knowledge into the periodic system.
Details
-
File Typepdf
-
Upload Time-
-
Content LanguagesEnglish
-
Upload UserAnonymous/Not logged-in
-
File Pages32 Page
-
File Size-