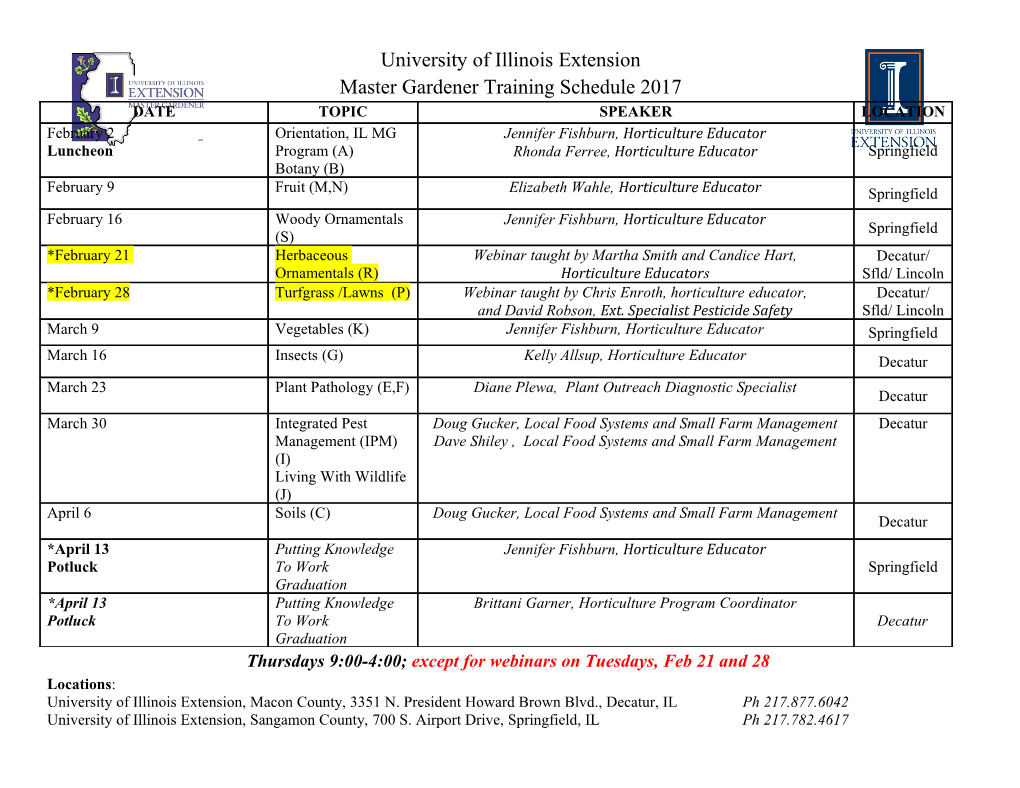
REMOTE RAMAN DETECTION OF NATURAL ROCKS A FINAL REPORT SUBMITTED TO THE DEPARTMENT OF GEOLOGY AND GEOPHYSICS, UNIVERSITY OF HAWAI’I AT MĀNOA, IN PARTIAL FULFILLMENT OF THE REQUIREMENTS FOR THE DEGREE OF MASTER OF SCIENCE IN GEOLOGY AND GEOPHYSICS August 2017 By Genesis Berlanga Advisor: Dr. Anupam K. Misra Remote Raman Detection of Natural Rocks 1,2* 1 1 GENESIS BERLANGA, TAYRO E. ACOSTA-MAEDA, SHIV K. SHARMA, JOHN N. 1 1 1,2 1 PORTER, PRZEMYSLAW DERA, HANNAH SHELTON, G. JEFFREY TAYLOR, 1 ANUPAM K. MISRA 1Hawai’i Institute of Geophysics and Planetology, University of Hawai’i, POST #602, 1680 East-West Road, Honolulu, Hawaii 96822, USA 2Department of Geology and Geophysics, University of Hawai’i, POST #702, 1680 East-West Road, Honolulu, Hawaii 96822, USA *Corresponding author: [email protected] Received XX Month XXXX; revised XX Month, XXXX; accepted XX Month XXXX; posted XX Month XXXX (Doc. ID XXXXX); published XX Month XXXX We report remote Raman spectra of natural igneous, metamorphic, and sedimentary rock samples at a standoff distance of 5 m. High quality remote Raman spectra of unprepared rocks are necessary for accurate and realistic analysis of future Raman measurements on planetary surfaces such as Mars. Our results display the ability of a portable Compact Remote Raman+LIBS+Fluorescence System (CRRLFS) to effectively detect and isolate various light and dark-colored mineral phases in natural rocks. The CRRLFS easily detected plagioclase and potassium feldspar end members, quartz, and calcite in rocks with high fluorescence backgrounds. Intermediate feldspars and quartz, when found in rocks with complex mineralogies, exhibited band shifts and broadening in the 504-510 cm-1 and 600-1200 cm-1 regions. A good approximation of intermediate plagioclase feldspars was possible by using spectral shape and assigning other minor Raman peaks in addition to the 504-510 cm-1 peaks. Detection of olivine and pyroxene in mafic rocks allowed for compositional characterization. OCIS codes: (120.0120) Instrumentation, measurement, and metrology; (220.0220) Optical design and fabrication; (280.0280) Remote sensing and sensors; (290.5860) Scattering, Raman; (300.6450) Spectroscopy, Raman; (300.6500) Spectroscopy, time-resolved. http://dx.doi.org/10.1364/AO.99.099999 1. INTRODUCTION (LIBS) measurements from standoff distances providing Raman spectroscopy is a notable active laser spectroscopy quantitative and qualitative elemental compositions of major technique that provides molecular and crystal arrangement and minor minerals on the surface of Mars [3]. This successful information about the target material enabling identification of laser operation on Mars has opened opportunities for both organics and inorganics. The technique involves shining complementary laser based techniques such as remote Raman light from a monochromatic laser, such as a 532 nm laser, on a spectroscopy, to provide additional mineralogical analyses. sample and collecting the resulting scattered light. A fraction of Raman spectroscopy can identify mineral structure, the scattered light is shifted in energy from the laser frequency distinguish between mineral polymorphs such as calcite and due to interactions between the incident electromagnetic aragonite, and the accompanying fluorescence signal can waves and the vibrational modes of the molecules in the identify biosignatures [4]. A combined approach of using time- sample. This inelastic shift called the Raman effect occurs resolved Raman along with LIBS and fluorescence effectively instantaneously at picosecond timescales of 10-12 s spectroscopy in one compact system, as seen in the future [1], [2]. A Raman spectrum is produced with band positions at Mars 2020 SuperCam instrument, will help in identifying frequencies corresponding to the energy levels of different various hydrated and biological minerals which will help in vibrational modes, univocally identifying a target sample [2]. NASA’s goal for search of life during planetary surface NASA’s Mars Science Laboratory (MSL) mission paved the explorations [4], [5]. way for active laser spectroscopy on a planetary surface. With this in mind, it is not surprising that time-resolved Curiosity Rover’s highly successful ChemCam instrument suite remote Raman spectroscopy is increasingly highlighted as a continues to acquire laser-induced breakdown spectroscopy viable method for planetary surface molecular analysis [4], [6]– [16]. This technique extends traditional micro-Raman Raman data from Mars using the SuperCam instrument on the techniques to standoff distances of up to hundreds of meters, Mars2020 mission. requires no sample preparation, causes minimal to no sample damage, and can be used under daylight conditions; saving time and increasing the number of accessible targets [7], [17]. The University of Hawai‘i (UH) has developed a Compact Remote Raman+LIBS+Fluorescence System (CRRLFS) that is capable of Raman, LIBS, and fluorescence measurements under daytime conditions from standoff distances of several meters [11]. The CRRLFS shown in Figure 1 is one of several in- house remote Raman systems developed for chemical and molecular analysis of liquid, gases, and solids, at distances between 1.5-430 m [18]–[25]. Studies by other laboratory groups have attempted to analyze various pure minerals, powders, and a selection of rocks using standoff and micro-Raman spectroscopy for planetary science applications. Micro-Raman systems use a very small laser spot size in the order of 10 µm in diameter for sample analysis. When studying rocks with multiple minerals, these systems normally give spectra of minerals that fall within the small laser spot size. Hence, it is difficult to identify a rock with a single micro-Raman measurement. To overcome this problem point-counting approaches are employed during which multiple spectra are measured at various parts of the rock to determine the presence of all mineral phases. In remote Raman systems a laser spot size of several millimeters is used Fig. 1. CRRLFS schematic (above) and actual system (below). and the Raman spectra obtained show the presence of all mineral phases falling within a much larger laser spot size. This The CRRLFS has been able to successfully acquire high technique provides bulk rock mineralogy. quality Raman spectra of various light and dark minerals, Previous micro-Raman studies have focused on point- water, water-ice, CO2 ice, and organic and inorganic chemicals counting approaches to accurately characterize bulk rock at distances of up to 50 m with a 10 s integration time [37]. In mineralogies [26]–[29], hand-held Raman studies illustrated this study several igneous, sedimentary, and metamorphic the possibility of effective Raman measurements in the field rocks were surveyed using the CRRLFS. We report accurate [8], [30], and remote Raman studies using fiber-coupled [4], and univocal identification of various mineral phases in natural [9], [22], [23], [31]–[33] and directly coupled optics and rock samples using the CRRLFS from a 5 m standoff distance spectrograph performed bulk sample analysis with using a 30 s integration time. X-ray diffraction (XRD) analyses centimeter-sized target areas at standoff distances [34]. were obtained for all samples to establish compositional Previous studies by the University of Hawai‘i at Mānoa Raman ground truth for comparison with the standoff Raman spectra. research group have demonstrated standoff Raman detection capability for a variety of minerals, rocks, and powder 2. METHODOLOGY chemicals using fiber-coupled optics and spectrograph [35]. Our directly coupled system design showed significant A. Samples improvement in remote Raman detection capability over the Twenty four rock samples were selected from Ward’s fiber-coupled system [36]. These directly coupled systems Collection of Classic North American Rocks 45-7250, Ward’s were developed in oblique geometries from 2-50 m [21], [37], Rock Forming Mineral Student Set 45 H 0355, and hand and also in coaxial geometries from 1.5-430 m [11], [18]–[20], samples from the Raman Spectroscopy Laboratory at the [24], [25], [36]. The advantages of the coaxial system over the University of Hawai‘i at Mānoa. Most rocks measured between oblique systems are large sampling depth and easy system 2-8 cm in length at their longest dimensions. Alkalic granite alignment. Previous work using coaxial systems was mostly (Quincy, MA, USA), nepheline syenite (Bancroft, Ontario, focused on obtaining remote Raman spectra of various Canada), tonalite (San Diego County, CA, USA), biotite gneiss minerals such as feldspars, hydrated minerals, dark colored (Uxbridge, MA, USA), grey sandstone (Berea, OH, USA), red minerals, various organic and inorganic chemicals, water, sandstone (Potsdam, NY, USA), quartz conglomerate (Fremont frost, ice, dry ice etc. However, to interpret in situ remote County, CO, USA), quartzite (Baraboo, WI, USA), pyroxenite Raman measurements from rocks and soils on planetary (harzburgite) (Stillwater Complex, MT, USA), and dunite surfaces such as Mars, standoff Raman measurements of (olivine peridotite) (Balsam, NC, USA) were chosen due to natural, unprepared rock samples are needed. These having more than one mineral component. Pink marble (Tate, measurements will be helpful in evaluating future remote GA, USA), dolomite marble (Essex County, Nj, USA), calcite (unknown locality), and siderite carbonatite (Iron Hill, CO, using a BNC Model 575 pulse-delay generator and custom USA) were chosen due to their systematic
Details
-
File Typepdf
-
Upload Time-
-
Content LanguagesEnglish
-
Upload UserAnonymous/Not logged-in
-
File Pages11 Page
-
File Size-