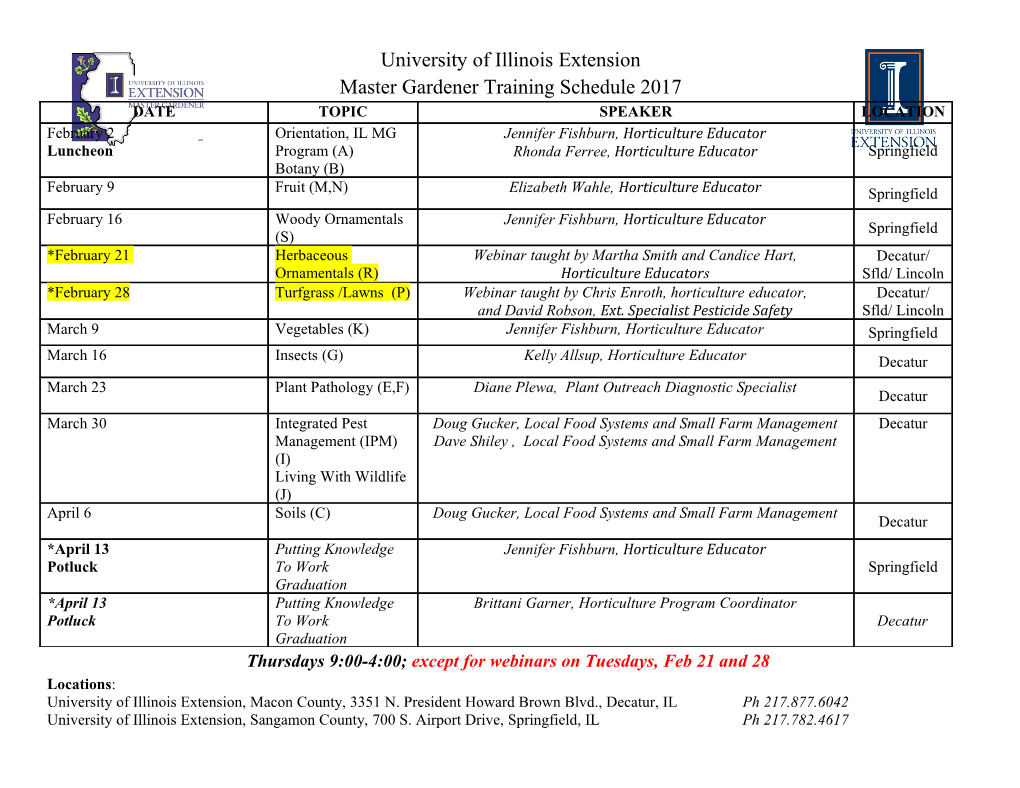
S´eminaire Poincar´e2 (2004) 1 – 16 S´eminaire Poincar´e 25 Years of Quantum Hall Effect (QHE) A Personal View on the Discovery, Physics and Applications of this Quantum Effect Klaus von Klitzing Max-Planck-Institut f¨ur Festk¨orperforschung Heisenbergstr. 1 D-70569 Stuttgart Germany 1 Historical Aspects The birthday of the quantum Hall effect (QHE) can be fixed very accurately. It was the night of the 4th to the 5th of February 1980 at around 2 a.m. during an experiment at the High Magnetic Field Laboratory in Grenoble. The research topic included the characterization of the electronic transport of silicon field effect transistors. How can one improve the mobility of these devices? Which scattering processes (surface roughness, interface charges, impurities etc.) dominate the motion of the electrons in the very thin layer of only a few nanometers at the interface between silicon and silicon dioxide? For this research, Dr. Dorda (Siemens AG) and Dr. Pepper (Plessey Company) provided specially designed devices (Hall devices) as shown in Fig.1, which allow direct measurements of the resistivity tensor. Figure 1: Typical silicon MOSFET device used for measurements of the xx- and xy-components of the resistivity tensor. For a fixed source-drain current between the contacts S and D, the potential drops between the probes P − P and H − H are directly proportional to the resistivities ρxx and ρxy. A positive gate voltage increases the carrier density below the gate. For the experiments, low temperatures (typically 4.2 K) were used in order to suppress dis- turbing scattering processes originating from electron-phonon interactions. The application of a 2 K. von Klitzing S´eminaire Poincar´e strong magnetic field was an established method to get more information about microscopic de- tails of the semiconductor. A review article published in 1982 by T. Ando, A. Fowler, and F. Stern about the electronic properties of two-dimensional systems summarizes nicely the knowledge in this field at the time of the discovery of the QHE [1]. Figure 2: Hall resistance and longitudinal resistance (at zero magnetic field and at B = 19.8 Tesla) of a silicon MOSFET at liquid helium temperature as a function of the gate voltage. The quantized Hall plateau for filling factor 4 is enlarged. Since 1966 it was known, that electrons, accumulated at the surface of a silicon single crystal by a positive voltage at the gate (= metal plate parallel to the surface), form a two-dimensional electron gas [2]. The energy of the electrons for a motion perpendicular to the surface is quantized (“particle in a box”) and even the free motion of the electrons in the plane of the two-dimensional system becomes quantized (Landau quantization), if a strong magnetic field is applied perpendicular to the plane. In the ideal case, the energy spectrum of a 2DEG in strong magnetic fields consists of discrete energy levels (normally broadened due to impurities) with energy gaps between these levels. The quantum Hall effect is observed, if the Fermi energy is located in the gap of the electronic spectrum and if the temperature is so low, that excitations across the gap are not possible. The experimental curve, which led to the discovery of the QHE, is shown in Fig. 2. The blue curve is the electrical resistance of the silicon field effect transistor as a function of the gate voltage. Since the electron concentration increases linearly with increasing gate voltage, the electrical resistance becomes monotonically smaller. Also the Hall voltage (if a constant magnetic field of e.g. 19.8 Tesla is applied) decreases with increasing gate voltage, since the Hall voltage is basically inversely proportional to the electron concentration. The black curve shows the Hall resistance, which is the ratio of the Hall voltage divided by the current through the sample. Nice plateaus in the Hall resistance (identical with the transverse resistivity ρxy) are observed at gate voltages, where the electrical resistance (which is proportional to the longitudinal resistivity ρxx) Vol. 2, 2004 25 Years of Quantum Hall Effect (QHE) 3 Figure 3: Copy of the original notes, which led to the discovery of the quantum Hall effect. The calculations for the Hall voltage UH for one fully occupied Landau level show, that the Hall 2 resistance UH /I depends exclusively on the fundamental constant h/e . becomes zero. These zeros are expected for a vanishing density of state of (mobile) electrons at the Fermi energy. The finite gate voltage regions where the resistivities ρxx and ρxy remain unchanged indicate, that the gate voltage induced electrons in these regions do not contribute to the electronic transport- they are localized. The role of localized electrons in Hall effect measurements was not clear. The majority of experimentalists believed, that the Hall effect measures only delocalized electrons. This assumption was partly supported by theory [3] and formed the basis of the analysis of QHE data published already in 1977 [4]. These experimental data, available to the public 3 years before the discovery of the quantum Hall effect, contain already all information of this new quantum effect so that everyone had the chance to make a discovery that led to the Nobel Prize in Physics 1985. The unexpected finding in the night of 4./5.2.1980 was the fact, that the plateau values in the Hall resistance ρxy are not influenced by the amount of localized electrons and can be expressed 4 K. von Klitzing S´eminaire Poincar´e 2 with high precision by the equation ρxy = h/ie (h=Planck constant, e=elementary charge and i the number of fully occupied Landau levels). Also it became clear, that the component ρxy of the resistivity tensor can be measured directly with a volt- and amperemeter (a fact overlooked by many theoreticians) and that for the plateau values no information about the carrier density, the magnetic field, and the geometry of the device is necessary. Figure 4: Experimental uncertainties for the realization of the resistance 1 Ohm in SI units and the determination of the fine structure constant α as a function of time. The most important equation in connection with the quantized Hall resistance, the equation 2 UH = h/e ·I, is written down for the first time in my notebook with the date 4.2.1980. A copy of this page is reproduced in Fig. 3. The validity and the experimental confirmation of this fundamental equation was so high that for the experimental determination of the voltage (measured with a x−y recorder) the finite input resistance of 1 MΩ for the x−y recorder had to be included as a correction. The calculations in the lower part of Fig. 3 show, that instead of the theoretical value of 25813 Ohm for the fundamental constant h/e2 a value of about 25163 Ohm should be measured with the x−y recorder, which was confirmed with high precision. These first measurements of the quantized Hall resistance showed already, that localized electrons are unimportant and the simple derivation on the basis of an ideal electron system leads to the correct result. It was immediately clear, that an electrical resistance which is independent of the geometry of the sample and insensitive to microscopic details of the material will be important for metrology institutes like NBS in the US (today NIST) or PTB in Germany. So it is not surprising, that discussions with Prof. Kose at the PTB about this new quantum phenomenon started already one day after the discovery of the quantized Hall resistance (see notes in Fig. 3). The experimental results were submitted to Phys. Rev. Letters with the title: “Realization of a Resistance Standard based on Fundamental Constants” but the referee pointed out, that (at this time) not a more accurate electrical resistor was needed but a better value for the fundamental constant h/e2. Interestingly, the constant h/e2 is identical with the inverse fine-structure constant −1 2 −7 2 α = (h/e )(2/µ0c) = 137.036 · · · where the magnetic constant µ0 = 4π10 N/A and the velocity of light c = 299 792 458 m/s are fixed numbers with no uncertainties. The data in Fig. 4 show indeed, that the uncertainty in the realization of the electrical unit of 1Ω within the International System of Units (SI units) was smaller (until 1985) than the uncertainty for h/e2 Vol. 2, 2004 25 Years of Quantum Hall Effect (QHE) 5 Figure 5: The number of publications related to the quantum Hall effect increased continuously up to a value of about one publication per day since 1995. or the inverse fine-structure constant. As a consequence, the title of the first publication about the quantum Hall effect was changed to: “New Method for High-Accuracy Determination of the Fine-Structure Constant Based on Quantized Hall Resistance”[5]. The number of publications with this new topic “quantum Hall effect” in the title or abstract increased drastically in the following years with about one publication per day for the last 10 years as shown in Fig. 5. The publicity of the quantized Hall effect originates from the fact, that not only solid state physics but nearly all other fields in physics have connections to the QHE as exemplarily demonstrated by the following title of publications: BTZ black hole and quantum Hall effects in the bulk/boundary dynamics [6]. Quantum Hall quarks or short distance physics of quantized Hall fluids [7]. A four-dimensional generalization of the quantum Hall effect [8]. Quantum computation in quantum-Hall systems [9]. Higher-dimensional quantum Hall effect in string theory [10]. Is the quantum Hall effect influenced by the gravitational field? [11].
Details
-
File Typepdf
-
Upload Time-
-
Content LanguagesEnglish
-
Upload UserAnonymous/Not logged-in
-
File Pages16 Page
-
File Size-