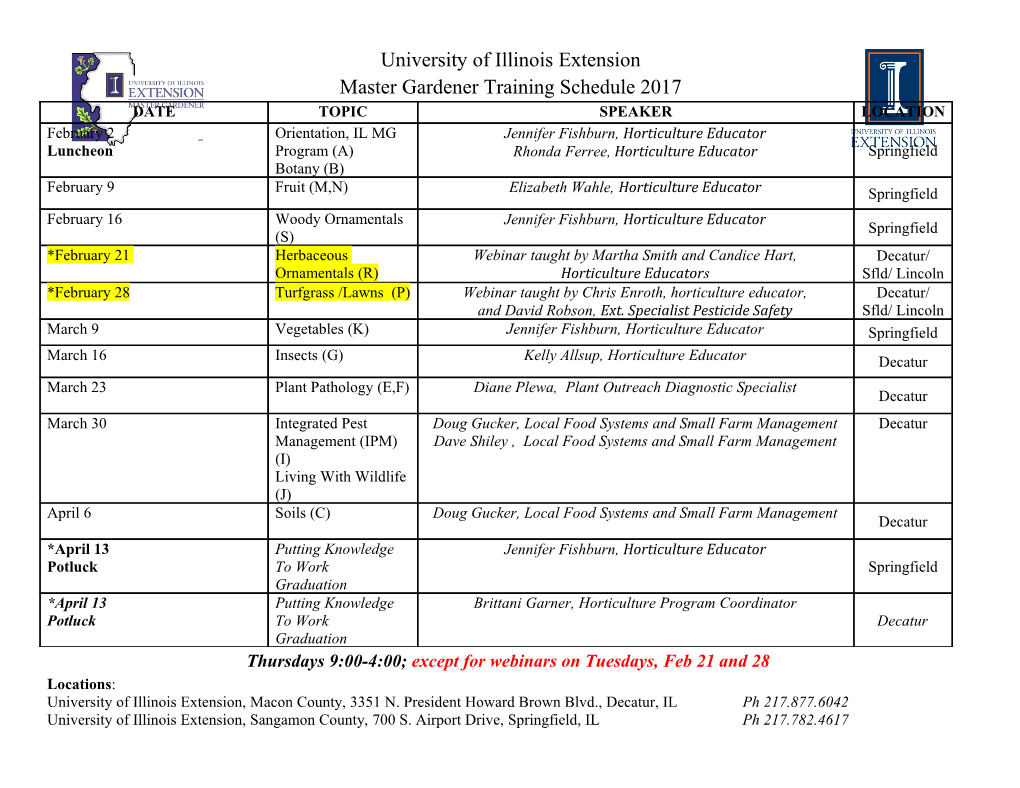
International Journal of Pure and Applied Mathematics Volume 119 No. 3 2018, 461-473 ISSN: 1311-8080 (printed version); ISSN: 1314-3395 (on-line version) url: http://www.ijpam.eu AP doi: 10.12732/ijpam.v119i3.6 ijpam.eu ON THE DISTRIBUTION OF THE RUNNING AVERAGE OF A SKELLAM PROCESS Weixuan Xia ∗Mathematical Finance Boston University Questrom School of Business 595 Commonwealth Ave, Boston, MA 02215, USA Abstract: It is shown that the probability distribution of the running average of a Skellam process is of compound Poisson type, which gives rise to double-uniform-sum distributions. The average process’s characteristic function, moments, as well as probability density function and cumulative distribution function are derived in explicit form. AMS Subject Classification: 60E10, 60G55, 60J75 Key Words: probability distribution, running average, Skellam process, double-uniform sum 1. Introduction A Skellam distribution is a discrete-valued probability distribution initially pro- posed in Skellam (1946) [6], and is well known to be the distribution of the difference of two independent Poisson random variables, with respective rate parameters λ > 0 and λ > 0. In continuous time t 0, a Skellam pro- 1 2 ≥ cess K (K ) is hence defined to be a L´evy process admitting a Skellam ≡ t distribution. In light of the conventional definition of L´evy processes (e.g., see Schoutens ((2003), pages 44–45) [5]), the Skellam process is defined by the following conditions. K = 0, a.s., i.e., Pr[K = 0] = 1. • 0 0 Received: May 18, 2107 c 2018 Academic Publications, Ltd. Revised: July 11, 2018 url: www.acadpubl.eu Published: July 12, 2018 462 W. Xia For any partition of time P = t N with t = 0 and t < t , • { n}n∈ 0 n n+1 n, it holds true that the increment random variables Ktn+1 Ktn are ∀ law − mutually independent and stationary with K K n = K n tn+1 − t tn+1−t ∼ Skellam(λ (t t ), λ (t t )). 1 n+1 − n 2 n+1 − n The mapping t R K Z is c`adl`ag with probability 1, i.e., • ∈ + 7→ t ∈ lim Pr[ K K > ǫ] = 0, ǫ > 0. hց0 | t+h − t| ∀ Conditional on t, the Skellam process has the following probability mass function, x/2 −(λ1+λ2)t λ1 pK|t(x) Pr[Kt = x] = e Ix(2t λ1λ2), x Z, (1) ≡ λ2 ∈ p where I ( ) is the modified Bessel function of the first kind. For details refer · · to Abramowitz and Stegun ((1972), pages 375–378) [1]. The characteristic function of K is hence given by φ (u) := E eiuKt eiuxp (x) (2) K|t ≡ K|t x∈Z X = exp λ t(eiu 1) + λ t(e−iu 1) , u R, 1 − 2 − ∈ where i = √ 1. Clearly, (2) is infinitely divisible in that φ (u) = (φ (u))t, − K|t K|1 so that the L´evy properties are meaningful. Several works have so far existed to discuss the properties as well as ap- plications of the Skellam process. For instance, Barndorff-Nielsen et al (2010) [2] considered the scaled Skellam process and a generalization using negative binomial distributions when modeling low-latency financial data while Kerss et al (2014) [4] analyzed, by means of time change, fractional Skellam processes of which the Skellam process is a special case. In this paper our interest lies in analyzing the following time-scaled integral of the path of the Skellam process, 1 t K˜ := K ds. (3) t t s Z0 This stochastic process, notably, can be identified as the running average of the Skellam process K. In equivalent differential, we can write 1 1 t dK˜ = K K ds dt, (4) t t t − t2 s Z0 ON THE DISTRIBUTION OF THE RUNNING... 463 with K˜0 = 0, a.s. This indicates that K˜ has continuous sample paths of bounded total variation. In the following sections the distributional information of K˜ is thoroughly explored, while comparison is also made with the original distribu- tional properties of K. 2. Characteristic function In general, the distribution of K˜ is analyzed conditional on t > 0 and the parametrization λ > 0, λ > 0 . In an attempt to derive the characteristic { 1 2 } function of K˜ , we introduce the following lemma, which applies quite conve- niently to the general class of L´evy processes. Lemma 1. If X (Xt) is a L´evy process and Y (Yt) its Riemann integral defined by ≡ ≡ t Yt := Xsds, (5) Z0 then it holds for the respective characteristic functions of X and Y that 1 φ (u) := E eiuYt = exp t ln φ (tuz)dz , u R. (6) Y |t X|1 ∈ Z0 Proof. By the independent and stationary increments of X, the decompo- sition n n t t Yt = lim Xkt/n = lim (n k + 1)(Xkt/n X(k−1)t/n) (7) n→∞ n n→∞ n − − Xk=1 Xk=1 immediately leads to n ktu φY |t(u) = exp lim ln φX|t/n , (8) n→∞ n ! Xk=1 from which the lemma follows by infinite divisibility. The next theorem hence gives the characteristic function of K˜ . Theorem 2. E iuK˜t φK˜ |t(u) := e (9) eiu 1 1 e−iu = exp λ t − 1 + λ t − 1 , u R. 1 iu − 2 iu − ∈ 464 W. Xia Proof. This follows from a direct application of Lemma 1 to (2) after scaling by 1/t. Notice that (9) has a removable singularity at u = 0, as it is easily ob- servable that the Taylor expansion of the function (ez 1)/z about 0 contains − all nonnegative integer-valued powers of z. Upon removal we can define that φ (0) = 1. As a consequence, K˜ ’s moment generating function, φ ( iu), K˜ |t K˜ |t − is uniformly well-defined on the real line, which allows the next section to ex- patiate on the moment properties. Obviously, like (2), (9) is still infinitely divisible. An important implication from (9) is that the running average process has a compound Poisson distribution, as stated below. Corollary 3. Nt law K˜t = Jn, (10) n=1 X where (Nt) is a Poisson process with intensity parameter λ1 + λ2 > 0 and J N are i.i.d. random variables admitting a double uniform distribution. { n}n∈ ++ Proof. Some elementary transformations from (9) lead to (eiu 1)e−iu(λ eiu + λ ) φ (u) = exp (λ + λ )t − 1 2 1 (11) K˜ |t 1 2 i(λ + λ )u − 1 2 λ eiu 1 λ 1 e−iu = exp (λ + λ )t 1 − + 2 − 1 , 1 2 λ + λ iu λ + λ iu − 1 2 1 2 which conveniently points to a compound Poisson structure with rate (λ1 +λ2)t. The mixing distribution is understood from −iu iu iuJ1 λ2 1 e λ1 e 1 φJ (u) := E e = − + − (12) λ1 + λ2 iu λ1 + λ2 iu to be a weighted average of the characteristic functions of two uniform distri- butions supported over [ 1, 0] and [0, 1], respectively. − In other words, the running average of the Skellam process is equivalent in law to a compound Poisson process with intensity λ1 +λ2 and double-uniformly distributed jumps. Nevertheless, the resulting distribution is no longer Skellam, in the absence of α-stability. ON THE DISTRIBUTION OF THE RUNNING... 465 3. Moments For succinctness, denote by mr the rth moment of the running average K˜ , with the definition r d φK˜ |t(u) m := E K˜ r = ( i)r . (13) r t − dur u=0 The uniform existence of the moments is as aforementioned, and they can be found by the following recursive formula. Theorem 4. r r λ + ( 1)k+1λ m = 1, m = t 1 − 2 m , r N. (14) 0 r+1 k k + 2 r−k ∈ Xk=0 Proof. Based on (9), the Taylor expansion of the characteristic exponent, ln φK˜ |t(u), around 0, gives that ∞ ∞ eiu 1 (iu)r 1 e−iu ( iu)r − = 1 + and − = 1 + − . (15) iu (r + 1)! iu (r + 1)! r=1 r=1 X X Then, we apply the famous exponential formula in combinatorics, a.k.a. Fa`a di Bruno’s formula in the context of exponentials (see Stanley ((1999), pages 1–10) [7]), in order to calculate the coefficients in ∞ m φ (u) = r (iu)r. (16) K˜ |t r! r=0 X As a result, r r λ t ( 1)k+1λ t m = (k + 1)! 1 + − 2 m , r N, (17) r+1 k (k + 2)! (k + 2)! r−k ∈ Xk=0 with m0 = 1, and the theorem follows. In connection with this, the mean, variance, skewness, and excess kurtosis can be calculated in proper order as (λ λ )t E[K˜ ] = m = 1 − 2 , (18) t 1 2 (λ + λ )t Var[K˜ ] = m m2 = 1 2 , (19) t 2 − 1 3 466 W. Xia 3 m3 3m2m1 + 2m1 3√3(λ1 λ2) Skew[K˜t] = − = − , (20) 3/2 3 m m2 4 (λ1 + λ2) t 2 − 1 2 4 ˜ m4 4m3m1 + 6m2m1 3m1 p 9 EKurt[Kt] = − 2 − 3 = . (21) m m2 − 5(λ1 + λ2)t 2 − 1 We remark that, compared to the Skellam process, E[K˜t]/E[Kt] = 1/2, Var[K˜t]/Var[Kt] = 1/3, Skew[K˜t]/Skew[Kt] = 3√3/4, 1 and EKurt[K˜t]/EKurt[Kt] = 9/5 . In comparison, the running average is char- acterized with smaller variance but higher asymmetric leptokurtic level. The mean and variance are still linear in time while the skewness and kurtosis gen- erally decrease with the passage of time.
Details
-
File Typepdf
-
Upload Time-
-
Content LanguagesEnglish
-
Upload UserAnonymous/Not logged-in
-
File Pages14 Page
-
File Size-