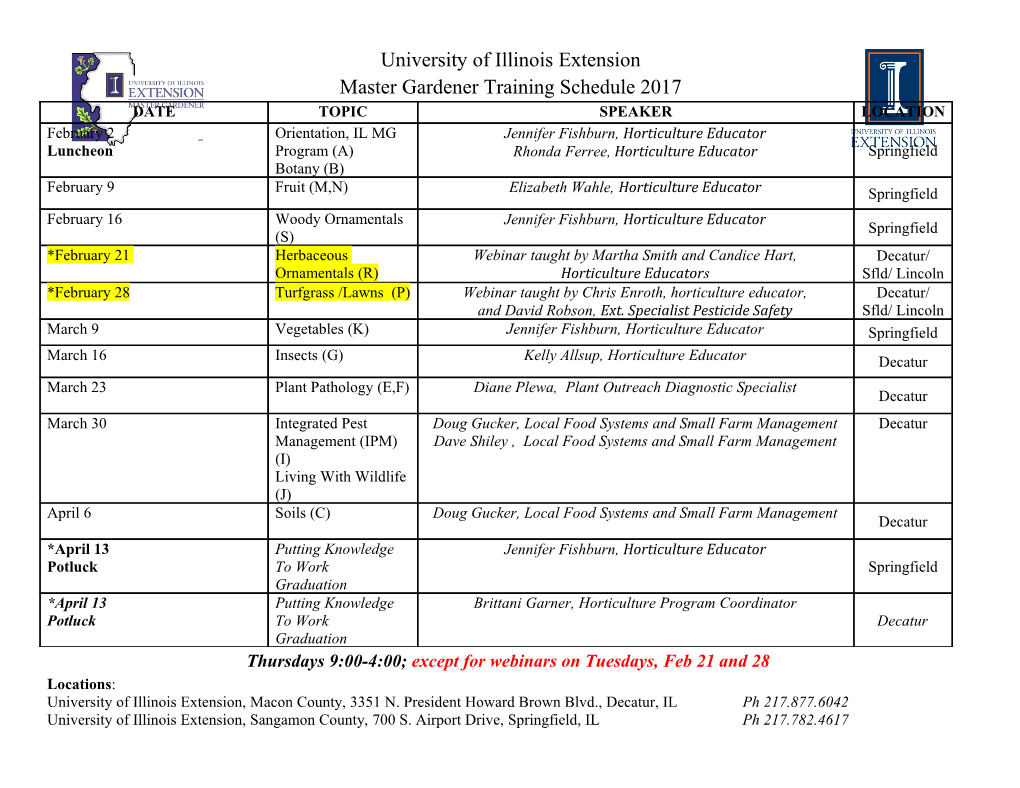
The Impact of Secondary Coordination Sphere Nucleophiles on Methane Activation: A Computational Study Mary E. Anderson* and Thomas R. Cundari* Contribution from the Department of Chemistry and Biochemistry Texas Woman’s University Denton, TX 76204 and Department of Chemistry Center for Advanced Scientific Computing and Modeling (CASCaM) University of North Texas Denton, TX 76203 *Corresponding Authors: [email protected], [email protected] 1 Abstract Density functional theory and ab initio calculations indicate that nucleophiles can significantly reduce enthalpic barriers to methane C–H bond activation. Different pieces of evidence point to an electrostatic origin for the nucleophile effect such as the sensitivity of the C–H activation barriers to the external nucleophile and to continuum solvent polarity. The data further imply a transition state with significant charge build-up on the active hydrogen of the hydrocarbon substrate. From the present modeling studies, one may propose proteins with hydrophobic active sites, available nucleophiles, and hydrogen bond donors as attractive targets for the engineering of novel methane functionalizing enzymes. TOC GRAPHIC H H d- E H C Nuc X H Active site polarity Hydrogen-bonding Outer-sphere nucleophile HB Activating atom Substituent effects Substrate KEYWORDS: catalysis, computational chemistry, methane activation, nucleophiles, outer coordination sphere, secondary coordination sphere. 2 Traditional catalyst design has focused on modulating activity and selectivity via manipulation of chemical groups that are directly – and typically strongly – bound to the active site, e.g., changing the steric and electronic profile of supporting ligands coordinated a metal catalyst.1 There is growing interest in the identification, quantification and exploitation of secondary (outer) sphere effects in catalysis. For example, Bronsted-Lowry acid/base conjugates,2 hydrogen-bonding,3 and redox inactive ions4 have been probed for their influence on catalytic active sites. Secondary sphere effects on transformations relevant to more efficient utilization of hydrocarbon-based resources such as natural gas and petroleum by selective transformations have seen less investigation.5 In general, chemical forces that may permit one to optimize catalyst performance via groups not directly bonded to the active site are weaker in a thermodynamic sense than the bonding interactions of covalently, ionically and datively coordinated groups directly bonded to a catalyst active site. The modeling of weaker secondary/outer sphere interactions– van der Waals, dispersion, hydrogen-bonding, electrostatics, etc. – remains a challenge for theory,6 particularly for the current workhorse of computational chemistry, density functional theory (DFT). Thus, continued testing and development of better theoretical protocols remains a critical need. To this end, a study was initiated of the impact of nucleophiles, simple models of those one might find in an enzyme active site, upon the activation of methane as a representative light alkane found in hydrocarbon feedstocks. Initial motivation for this research came from classical valence bond (VB) computations, which indicate a surprisingly large contribution from ionic resonance structures to the ground state wavefunction of methane.7,8 In a study by Hiberty and Cooper, in which a single C–H bond of methane was correlated, it was found that the covalent (C–H) resonance structure comprised ~70% of the ground state description of methane, with near equal contributions from hydridic (C+H-) and protic (C-H+) resonance structures.7 In a VB study of 3 methane in which all 4 C–H bonds are correlated, Karplus and coworkers found that bi-ionic + - resonance structures (e.g., C(–H)2(H )(H )) also made important contributions to the ground state bonding of methane.8 Research on methane activation indicates that the acid/base properties of methane are critically important in the identification of catalyst leads with sufficient activity to both activate and functionalize methane. The classic work of the Olah (superacids9) and Streitwieser (superbases10) groups have shown that with sufficiently reactive reagents even methane can be made to reveal its deeply repressed Brønsted-Lowry acid/base tendencies. However, superacid and superbase systems are typically stoichiometric, require forcing conditions, and/or exotic reagents. For example, Olah and coworkers showed that methane can be converted to methanol, but this required elevated temperatures, the strongest superacids, 100% hydrogen peroxide; conversion rates and selectivity were also less than desirable for a practical catalyst.11 One may propose that modulation of the acid/base properties of methane through both primary and secondary coordination sphere effects is a reasonable target in identifying catalyst leads that can both activate and functionalize light alkane C–H bonds without the need to resort to superacidic/basic active site functionalities. Modelling the active site of ethylbenzene dehydrogenase (EBDH), which selectively hydroxylates a benzylic C–H bond of ethylbenzene, calculations by Nazemi et al.,12 in conjunction with pioneering experimental and computational work by the Heider and Szalenic groups,13 point to a transition state for C–H activation with considerable protic character (viz … … … R H O Mo Ln; R = hydrocarbyl, Ln = supporting ligands) even though the reaction entails formal transfer of a hydrogen atom. The activity of EBDH is thus moderated to a significant extent by an active site histidine.12-14 Further research suggests that hydrogen bonding and the 4 electrostatic stabilization afforded by a protonated amine are also important factors in controlling the reactivity of EBDH.12,14 In the present Letter the focus is on nucleophiles, simple models of those one might find in a biological milieu, and calculating their impact upon the activation barriers for methane C–H activation. Our hypothesis was that in light of valence bond studies of methane7,8 and earlier work on the acid/base properties of hydrocarbon C–H bonds,12-14 secondary coordination sphere nucleophiles would stabilize transition states for aliphatic C–H bond activation, thus reducing the intrinsic barrier to this critical step in the catalytic cycle for methane functionalization. Model Selection. As our interest is in the activation of light alkanes, methane is the obvious target substrate. Methane has a homolytic C–H bond dissociation enthalpy (BDE) of 105 kcal/mol.15 A neutral, radical entity was sought for these studies – one with an electronegative activating atom as a simple, yet chemically reasonable model of organic and biological C–H activators – with a O–H BDE that is neither too high (typical of very potent oxidants such as hydroxyl radical, BDEOH ~ 120 kcal/mol) nor too low (e.g., stable nitroxyl radicals such as 15 • TEMPO, BDEOH ~ 70 kcal/mol). Using these criteria, the hydroperoxyl radical (HOO ) was 15 chosen as a model activator, BDEO—H ~ 88 kcal/mol. Additionally, for the present study, such models afforded the opportunity to assess the accuracy and precision of the calculations through comparison of density functional and high accuracy ab initio techniques. Researchers have estimated that the effective dielectric constants of protein active sites can very over a very wide range from very hydrophobic to highly hydrophilic.161718 With the expected sensitivity of secondary coordination sphere effects to solvent environment, a continuum solvent of medium polarity, tetrahydrofuran (THF), was initially modeled within the framework of the 5 SMD model. Further examination of the influence of the polarity of the continuum solvent is presented below. Given the aforementioned selections, the baseline system for this study is defined by the reaction, • + … … ‡ • HOO H—CH3 [HOO H CH3] HOOH + CH3 M06-L/6-311++G(d,p) calculations yield a computed enthalpic barrier for hydrogen atom abstraction (HAA) of H‡ = +22.9 kcal/mol. The DFT-calculated transition state geometry for methane activation by hydroperoxyl radical is given in Figure 1A (next page; upper left hand corner). The computed methane activation barrier was only slightly higher using M06-L in conjunction with correlation consistent basis sets up to quintuple-zeta quality (cc-pVZ, = D, T, Q, 5), H‡ = +23.9 ± 0.4 kcal/mol. Using the M06-L/6-311++G(d,p) calculated geometries and enthalpic corrections, CCSD(T)/cc-pVZ simulations give calculated barriers for the above reaction of 26.9 ( = D), 24.3 ( = T), 23.7 ( = Q), and 23.4 ( = 5) kcal/mol, in excellent agreement with the DFT-predicted barrier of 22.9 kcal/mol. A B 6 C D E F G H Figure 1. M06-L/6-311++G(d,p)-calculated transition state geometries for the activation of methane by hydroperoxyl radical in the presence of different nucleophiles. A: no nucleophile, baseline system; B: methylthiol; C: methylselenate; D: methylthiolate; E: hydroxide; F: chloride; G: formate; H: bromide; I: fluoride . Bond lengths in Å; bond angles in degrees. 7 Table 1. M06-L/6-311++G(d,p)/SMD-THF-calculated enthalpic barriers for methane activation by hydroperoxyl radicals in the presence of external nucleophiles Nuc. H‡ (kcal/mol) -- 22.9a MeS- 15.5 MeSH 22.4 F- 22.0 Cl- 21.6 Br- 19.0 HO- 13.1 - HCO2 20.4 MeSe- 16.2 aBaseline system with no nucleophile (see Figure 1A). Scheme 1. Depiction of transition states for hydrogen atom abstraction of methane by hydroperoxyl radical in the presence of different nucleophiles (X). 8 Impact of Nucleophiles. Table 1 organizes the
Details
-
File Typepdf
-
Upload Time-
-
Content LanguagesEnglish
-
Upload UserAnonymous/Not logged-in
-
File Pages15 Page
-
File Size-