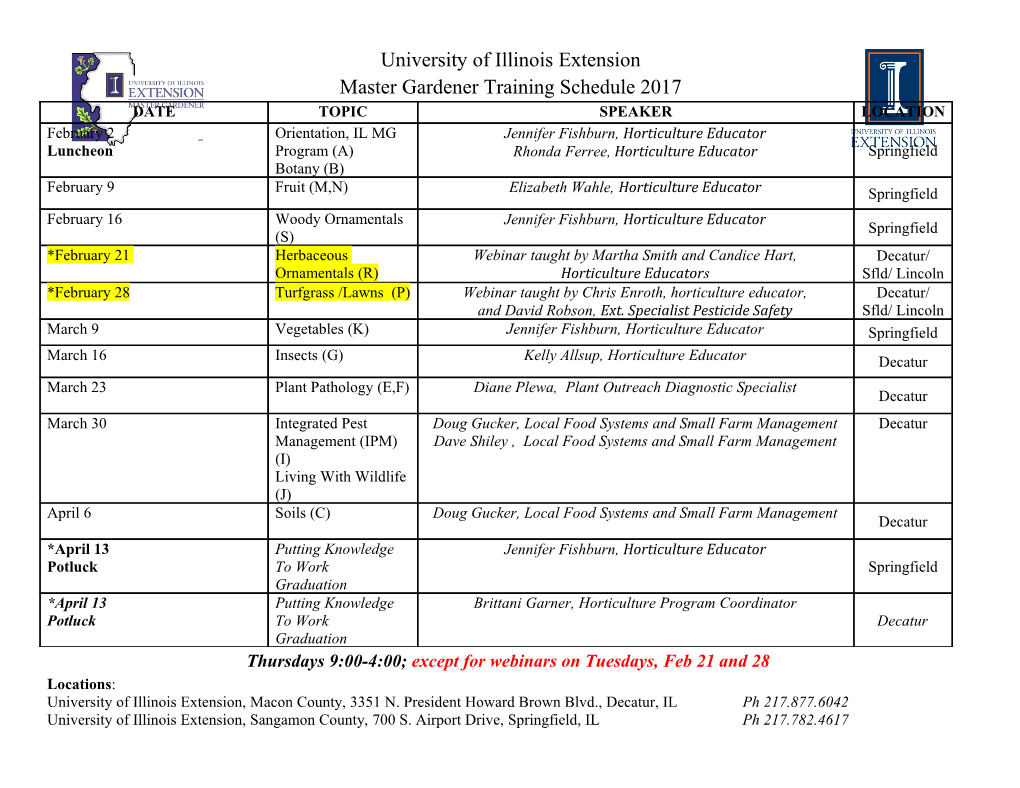
BOREL SETS AND FUNCTIONS IN TOPOLOGICAL SPACES JIRˇ´I SPURNY´ Abstract. We present a construction of the Borel hierarchy in general topo- logical spaces and its relation to Baire hierarchy. We define mappings of Borel class α, prove the validity of the Lebesgue–Hausdorff–Banach characterization for them and show their relation to Baire classes of mappings on compact spaces. Obtained result are used for studying Baire and Borel order of com- pact spaces, answering thus one part of the question asked by R.D. Mauldin. We present several examples showing some natural limits of our results in non-compact spaces. 1. Introduction The Borel hierarchy is a classical topic deeply studied within the framework of metrizable spaces, we refer the reader to [1], [31], [18], [6], [46] and [28] for detailed surveys of results in separable metrizable spaces and [48], [13], [14], [22] and [21] for results in non-separable metrizable spaces. On the other hand, the question how to develop a satisfactory theory of Borel classes in general topological spaces have been studied much less extensively (see e.g. [8], [19], [20], [16], [17], [40], [41] and [23]). The aim of our paper is to present an attempt in this direction. As one expects, we often use well known methods. We have tried to quote all sources known to us that are needed and/or closely related to our work. However, because of the vast amount of work in this area we have not attempted to collect all the references connected with this topic. Since it requires some effort to introduce all notions, we postpone the definitions and results to the relevant sections and just briefly describe the content of the paper. We start by the introduction of Borel classes defined in an abstract way from a general family of sets (see Definition 2.1). Then we introduce abstract classes of mappings defined as pointwise limits from a given family of mappings and prove the Lebesgue–Hausdorff–Banach characterization of these mappings via the preimages of open sets (see Theorem 2.7). The main Section 3 introduces classes of Baire, Borel and resolvable sets in topo- logical spaces. We show their relation to the classical Borel hierarchy in metrizable spaces (Proposition 3.4). The main result formulated in Theorem 3.6 shows coin- cidence of all defined classes for Baire sets in compact spaces. A key method used in the proof of Theorem 3.6 is the selection lemma [23, Lemma 1]. We finish the 2000 Mathematics Subject Classification. 54H05, 28A05. Key words and phrases. Baire sets, Borel sets, H–sets, Baire functions, Borel functions, Baire and Borel order, scattered–K–analytic, Cechˇ analytic and K–analytic spaces, functions of the first Baire and Borel class. The work is a part of the research project MSM 0021620839 financed by MSMT and partly supported by the grants GA CRˇ 201/06/0018, and GA CRˇ 201/07/0388. 1 2 JIRˇ´I SPURNY´ section by presenting a result on “absoluteness” of Baire classes in Theorem 3.7 (cf. [23, Theorem 5]). Results of Section 3 are used in Section 4 for studying order of Baire, Borel and resolvable sets in topological spaces. Theorem 4.2 answers one part of the question asked by R.D. Mauldin in [34, Question, p. 440] and [35, Problem, p. 395]. The next section starts by proving the Lebesgue–Hausdorff–Banach characteriza- tion of mappings on compact spaces (Theorem 5.2). Then we prove in Corollary 5.6 the equality between Baire measurable mappings and mappings of some Baire class for mappings with values in arcwise connected locally arcwise connected metric spaces and provide several characterizations of mappings of the first Baire class on compact spaces (Theorem 5.8). Several examples and questions finish the paper. Let us recall several definitions. If X is a set and F is a family of its subsets, then F is a sublattice, if ∅, X ∈ F and F is closed with respect to finite unions and intersections. The family F is an algebra if F is a sublattice that is closed with respect to complements. If F is a family of sets in a set X, we write Fσ (respectively Fδ) for all countable unions (respectively intersections) of sets from F. We write χA for the characteristic function of a set A and f|A for the restriction of a function f on A. If f : X → Y is a mapping from X to a topological space Y , we say that f is F–measurable, if f −1(U) ∈F for each open U ⊂ Y . All topological spaces are considered to be Tychonoff (see [4, p. 39]. 2. Generation of sets and functions Definition 2.1. If F is a family of sets in a set X, we define Borel classes generated by F as follows: Let Σ1(F) = F, Π1(F) = {X \ F : F ∈ F}, and for α ∈ (1, ω1), let Σα(F) = Πβ(F) σ β<α [ and Πα(F) = Σβ(F) . δ β<α [ The family Σα(F) is termed the sets of additive class α, the family Πα(F) is called the sets of multiplicative class α. The sets in ∆α(F) = Σα(F) ∩ Πα(F) are the sets of ambiguous class α. Remark 2.2. The definition and notation of abstract Borel classes is chosen in such a way that it coincides with the definition of Borel sets in metrizable spaces, see [28, Section 11.B] and Proposition 3.4. The following proposition collects facts on abstract Borel classes that one expects to hold (see [28, Section 11.B, Exercise 22.17 and Proposition 22.15] or [31, §26]). Proposition 2.3. Let F be an algebra of sets in a set X. Then the following assertions hold: (a) Σα(F) ∪ Πα(F) ⊂ ∆β(F), 0 < α < β < ω1, (b) a set A ⊂ X is in Σα(F) if and only if X \ A ∈ Πα(F), α ∈ (0, ω1), (c) the family Σα(F) is stable with respect to countable unions and finite in- tersections, Πα(F) is stable with respect to finite unions and countable in- tersections and ∆α(F) is an algebra, α ∈ (1, ω1), BOREL SETS AND FUNCTIONS IN TOPOLOGICAL SPACES 3 (d) α<ω1 Σα(F) = α<ω1 Πα(F) is the σ–algebra generated by F, (e) Σα(F) = (∆α(F))σ, α ∈ (1, ω ), S S 1 (f) for each α ∈ (1, ω1), Σα(F) has the generalized reduction property, that is, ′ if An ∈ Σα(F), n ∈ N, then there exist pairwise disjoint sets An ∈ Σα(F), ′ ∞ ′ ∞ n ∈ N, such that An ⊂ An and n=1 An = n=1 An, (g) if α ∈ (1, ω1) and A ∈ ∆α+1(F), then there exists a sequence {An} of sets S S such that An ∈ ∆α(F), n ∈ N, and χA = lim χAn , (h) if α ∈ (1, ω1) is limit and A ∈ ∆α+1(F), then there exists a sequence {An} of sets such that An ∈ β<α ∆β(F), n ∈ N, and χA = lim χAn . Proof. Assertions (a), (b) and (c)S follow by a straightforward transfinite induction, (d) follows from (a) and (c), and (e) follows from (a). For the proof of (f), let An ∈ ∞ Σα(F), n ∈ N, be given. Using (e) we write An = k=1 Ank, where Ank ∈ ∆α(F). ′ We enumerate the family {Ank : n, k ∈ N} as {B } and define j S ′ ′ ′ ′ B1 = B1, Bj = Bj \ (B1 ∪ · · · ∪ Bj−1), j ≥ 2. Then the sets Bj are contained in ∆α(F). We set ′ A1 = {Bj : Bj ⊂ A1} and ′ [ ′ ′ An = {Bj : Bj ⊂ An,Bj * A1 ∪ · · · ∪ An−1}, n ≥ 2. ′ [ ′ ′ Then An ⊂ An and An ∈ Σα(F), n ∈ N. Moreover, {An : n ∈ N} is a disjoint ∞ family whose union equals to n=1 An. To verify (g), let A ∈ ∆α+1(F) for some α ∈ (1, ω1) be given. By the definition, ∞ ∞ S A = n=1 Bn = n=1 Cn, where Bn ∈ Πα(F), Cn ∈ Σα(F), n ∈ N. We fix n ∈ N and use (f) for the couple {X \ Bn, Cn} to find a set An ∈ ∆α(F) such that S T Bn ⊂ An ⊂ Cn. Then lim χAn = χA, as required. For the proof of (h), let α be a countable limit ordinal and A ∈ ∆α+1(F). We ∞ ∞ write A = n=1 Bn = n=1 Cn, where Bn ∈ Πα(F), Cn ∈ Σα(F), n ∈ N. By (a), there exists ordinals αnk < α and sets Bnk, Cnk ∈ ∆αnk (F) so that S T ∞ ∞ Bn = Bnk and Cn = Cnk, n, k ∈ N. k k \=1 [=1 Without loss of generality we may assume that the sequences {Bn}, {Cnk} are increasing and the sequences {Cn}, {Bnk} are decreasing. Then the sets n p An = (Bpn ∩ Cjn), n ∈ N, p=1 j=1 [ \ are contained in ∆αn (F) for some αn < α and lim χAn = χA (see [34, proof of Theorem 2.1]). This concludes the proof. Definition 2.4. If Φ is a family of mappings from a set X to a topological space Y , inductively we define Baire classes generated by Φ as follows: Let Φ0 = Φ and for each countable ordinal α ∈ (0, ω1), let Φα be the family of all pointwise limits of sequences from β<α Φβ. S 4 JIRˇ´I SPURNY´ Remark 2.5. Later on (see Theorem 2.7 and Definition 5.1), it will be sometimes convenient to denote the starting family of the inductive definition as Φ1. More precisely, we start from a family denoted as Φ1 and then Φα consists of all pointwise limits of sequences from 1≤β<α Φβ, α ∈ (1, ω1).
Details
-
File Typepdf
-
Upload Time-
-
Content LanguagesEnglish
-
Upload UserAnonymous/Not logged-in
-
File Pages18 Page
-
File Size-