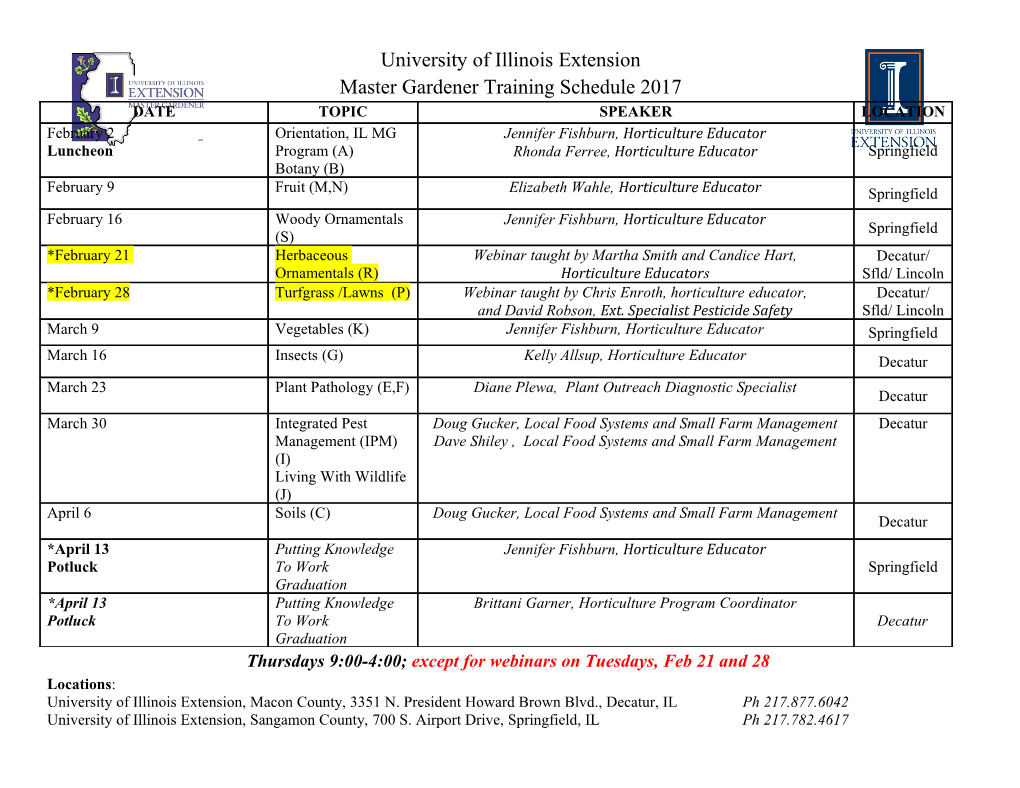
Lab Notes Editors Thomas M. Moses | Shane F. McClure Resin-Coated and Clarity-Enhanced AQUAMARINE Pendant The Carlsbad laboratory received a 34.24 ct light greenish blue free-form pendant (figure 1) measuring 33.40 × 25.75 × 6.40 mm. One side of the pen- dant was flat and polished, and the other side had a rough surface. Stan- dard gemological testing gave a refrac- tive index (RI) of 1.570–1.580. The pendant had the same reaction to long-wave and short-wave UV light: blue fluorescence in the center and green fluorescence around the edges of the stone. Examination with a stan- dard gemological microscope revealed a blue and orange flash effect most clearly visible from the flat side (fig- ure 2, left), which is diagnostic of clar- ity enhancement. Ultraviolet/visible/ near-infrared (UV-Vis-NIR) spectra were consistent with natural aquama- rine, with absorption peaks at 370 and 425 nm and an absorption band at ~830 nm (figure 2, right). Raman spec- troscopy showed the presence of poly- mer on the rough side of the stone and aquamarine on the polished flat side. Although emeralds are the variety of beryl most commonly associated with clarity enhancement, filled aquamarine has previously been re- Figure 1. This 34.24 ct light greenish blue free-form aquamarine pendant, ported in the Chinese market (J. Li et measuring 33.40 × 25.75 × 6.40 mm, was seen in the Carlsbad laboratory. al., “Polymer-filled aquamarine,” Fall Stone courtesy of Stephen Challener. 2009 G&G, pp. 197–199). Only one side, the rough side, was higher luster than the polished flat Editors’ note: All items were written by staff coated with polymer resin. Viewed in side. Fourier-transform infrared (FTIR) members of GIA laboratories. the microscope, this side appeared to spectroscopy taken from the rough have a thin transparent layer on the side revealed strong diagnostic peaks EMS EMOLOGY G & G , Vol. 55, No. 2, pp. 246–259. surface. Trapped air bubbles were vis- at ~3100–2850 cm–1 (figure 3, right). © 2019 Gemological Institute of America ible with magnification (figure 3, left), Although clarity enhancement and areas with the coating had a and coating of beryl gemstones are 246 LAB NOTES GEMS & GEMOLOGY SUMMER 2019 UV-VIS-NIR SPECTRUM 10 9 8 7 6 5 4 3 2 ABSORBANCE (ARB. UNITS) ABSORBANCE 1 0 330 430 530 630 730 830 930 WAVELENGTH (nm) Figure 2. Left: The orange and blue flash effect seen in a clarity-enhanced fracture of the aquamarine; field of view 4.24 mm. Right: The UV-Vis-NIR spectrum, with absorption peaks at 370 and 425 nm and an absorption band at ~830 nm, is consistent with natural aquamarine. not uncommon, this material is rarely diamond lattice when the material is radiation; green stains are commonly seen in a gemological laboratory. Both hundreds of kilometers deep in the associated with naturally colored treatments are readily identified, but earth’s mantle. But most natural green diamonds. careful examination of every stone is green diamonds are colored by radia- Recently, GIA’s Carlsbad labora- important to identify them. tion damage that occurs at very shal- tory examined an interesting attempt Michaela Stephan low depths in the earth’s crust over to imitate a natural green diamond long periods of time. Commonly ac- when a 6.49 ct green crystal was sub- companying this exposure to radioac- mitted for a Colored Diamond Grad- tive minerals and fluids are surface ing Report (figure 4). The surfaces of Rough DIAMOND Crystal with an patches of green or brown color that the resorbed octahedron were covered Unusual Coating of Fake Green gemologists call “radiation stains.” by uneven patches of green color. “Radiation Stains” These are simply areas of extreme Fourier-transform infrared (FTIR) Due to their rare occurrence and the damage to the diamond structure analysis revealed a typical type Ia dia- environment required to create their caused by alpha particle radiation. mond with abundant nitrogen and hy- color, natural green diamonds are Initially green, they turn brown im- drogen impurities. However, the some of the world’s most beautiful mediately after exposure to tempera- ultraviolet-visible (UV-Vis) spectrum and sought-after gems. Most dia- tures above 500°C. These surface was very unusual and did not show monds acquire their color from impu- features indicate that a diamond has any of the bands that are produced by rities or defects incorporated into the been exposed to a source of natural radiation damage in diamond (e.g., the Figure 3. Left: Gas bubbles and high luster on the aquamarine’s resin-coated rough surface, shown near the drill hole. Field of view 7.19 mm. Right: The FTIR spectrum shows polymer peaks at ~3100–2850 cm–1. The dotted line is the spectrum of an untreated aquamarine, shown for reference. FTIR SPECTRA ' '' polymer ' t '' / ' ' '' '' ',..'\, A ._,., I _, _____ • ABSORBANCE ABSORBANCE , ..--------"' 4200 4000 3800 3600 3400 3200 3000 2800 2600 WAVENUMBER (cm–1) LAB NOTES GEMS & GEMOLOGY SUMMER 2019 247 RAMAN SPECTRUM 12000 1332 11000 552 10000 9000 8000 613 INTENSITY (COUNTS) (COUNTS) INTENSITY 7000 1400 1200 1000 800 600 400 RAMAN SHIFT (cm–1) Figure 6. The Raman spectrum (514 nm laser excitation) of the rough dia- mond’s green coating shows clear peaks from chromium oxide (552 and 613 cm–1) as well as the underlying diamond (1332 cm–1). The specific po- sitions of the chromium oxide peaks suggest the coating has been an- Figure 4. This 6.49 ct rough dia- nealed to ~700°C (Mohammadtaheri et al., 2018). mond with patchy green surface color was submitted to GIA’s Carlsbad laboratory for a Col- ored Diamond Grading Report. face (figure 5). Individual platy crys- sis identified the green plates as tals could easily be flaked off using chromium oxide (figure 6). The tweezers or a pointer probe, but they Raman peak positions correlated very GR1 defect). Careful examination remained adhered to the surface well with chromium oxide powder under magnification found that the under normal gem testing situations, that had been heated to ~700°C to green color was not due to radiation including wiping the diamond with a produce a crystallized coating (M. stains, but instead to groupings of stone cloth. X-ray fluorescence (XRF) Mohammadtaheri et al., “The effect emerald-green platy crystals, ~40 μm analysis indicated high concentra- of deposition parameters on the struc- in size, attached to the diamond sur- tions of chromium, and Raman analy- ture and mechanical properties of chromium oxide coatings deposited by reactive magnetron sputtering,” Figure 5. The diamond’s patchy green coloration is caused by interlocking Coatings, Vol. 8, No. 3, 2018, Article platy crystals of chromium oxide, visible with magnification. Field of No. 111). A small H1b defect present view 0.72 mm. in the diamond’s FTIR spectrum sup- ports this annealing temperature. Most colored coatings encountered in the lab are on faceted stones and are pink, orange, red, or blue. The warmer hues are typically created by sputter- coating techniques involving metals and the colder hues by inks or other or- ganic dyes. The use of chromium oxide powder to produce a green coating— along with annealing of the powder to produce crystallized plates that adhere to the rough surface to resemble radia- tion stains—represents a significant at- tempt to artificially reproduce the features seen on natural green dia- monds. While the unique coating is easily discerned from natural green ra- diation stains under magnification, this stone is a strong reminder to care- fully examine any green diamond, 248 LAB NOTES GEMS & GEMOLOGY SUMMER 2019 Figure 7. The two stones on the far left, corresponding to the blue analytical points in figure 8, were classified as kornerupine. The center 20 stones, corresponding to the yellow analytical points in figure 8, were classified as pris- matine. For the eight stones on the right, corresponding to the red analytical points, the species could not be deter- mined. The species classifications of all the stones were based on chemistry acquired by LA-ICP-MS. The largest stone is the 11.9 ct brown oval at the top of the center group. The smallest is the light blue cushion stone at the bottom of the center group, weighing 0.93 ct. even rough crystals, in order to know rich compositions in the kornerupine mining gem tourmaline species by LA- exactly what you are buying. group,” Mineralogical Magazine, Vol. ICP-MS,” Spring 2019 G&G, pp. 2–17). Virginia A. Schwartz and 60, No. 400, 1996, pp. 483–491). As a result, we have slightly modified Christopher M. Breeding GIA laboratories have recently im- the criteria for separating the two to plemented a method using laser abla- account for analytical error. If the tion–inductively coupled plasma–mass boron apfu is between 0 and 0.45, the spectrometry (LA-ICP-MS) to quantify stone is classified as kornerupine. Be- Separation of KORNERUPINE and boron concentration in kornerupine tween 0.45 and 0.55 apfu, the species PRISMATINE and prismatine in order to distinguish cannot be determined by this method. Kornerupine and prismatine form a the two. The 30 stones shown in figure If the boron apfu is between 0.55 and solid solution series with a general 7—all thought to be kornerupine— 1, the stone is classified as prismatine. chemical formula of X( ,Mg,Fe) were borrowed from the GIA Museum For three spot analyses, if one spot has M(Al,Mg,Fe) T(Si,Al,B) (O,OH,F) (X: and tested using a Thermo Fisher a boron apfu between 0.45 and 0.55, 9 5 □ 22 cubic site; M: octahedral sites; T: tetra- iCAP Qc ICP-MS, coupled with an Ele- the species is undetermined.
Details
-
File Typepdf
-
Upload Time-
-
Content LanguagesEnglish
-
Upload UserAnonymous/Not logged-in
-
File Pages14 Page
-
File Size-