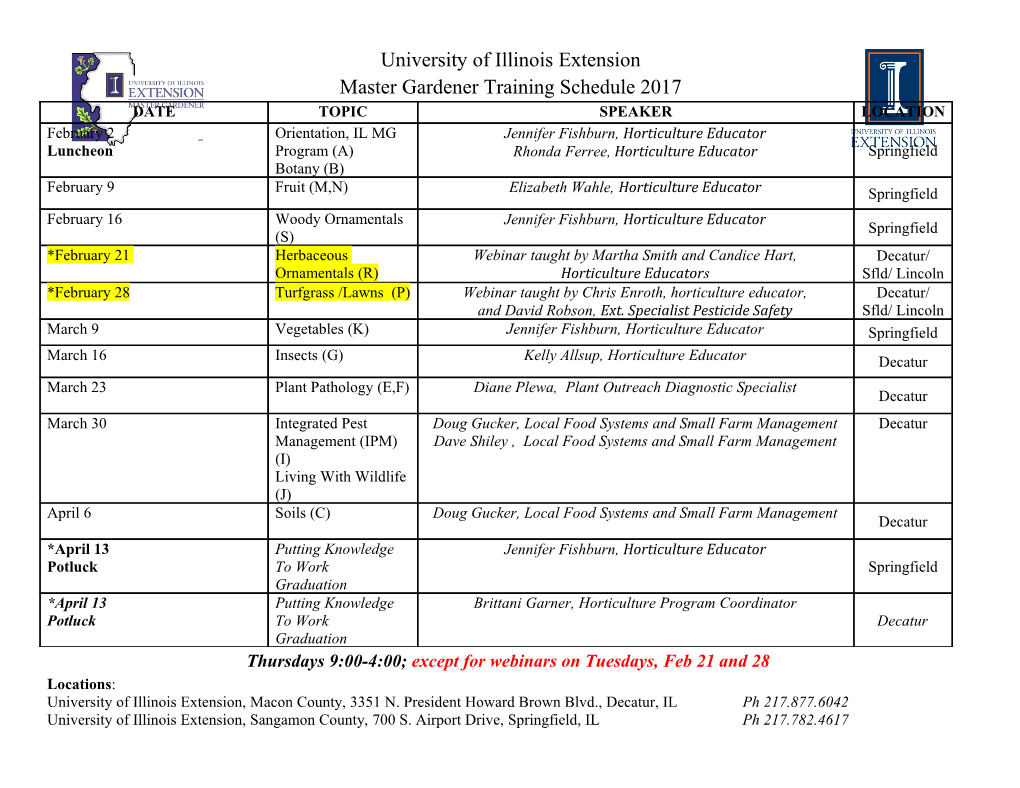
SLAC{PUB{7564 Princeton/HEP/97{8 UR{1501 UTKHEP{00010 June 1997 Positron Pro duction in Multiphoton Light-by-Light Scattering D.L. Burke, R.C. Field, G. Horton-Smith, T. Kotseroglou, J.E. Sp encer and D. Walz Stanford Linear Accelerator Center, Stanford University, Stanford, California 94309 S.C. Berridge, W.M. Bugg, K. Shmakov and A.W. Weidemann DepartmentofPhysics and Astronomy, UniversityofTennessee, Knoxville, Tennessee 37996 C. Bula, K.T. McDonald and E.J. Prebys Joseph Henry Lab oratories, Princeton University, Princeton, New Jersey 08544 y z x C. Bamb er, S.J. Bo ege, T. Ko as, A.C. Melissinos, D.D. Meyerhofer, D.A. Reis and { W. Ragg DepartmentofPhysics and Astronomy, UniversityofRochester, Ro chester, New York 14627 Abstract A signal of 106 14 p ositrons ab ove background has b een observed in collisions of a low-emittance 46.6-GeV electron b eam with terawatt pulses from a Nd:glass laser at 527 nm wavelength in an exp eriment at the Final Fo cus Test Beam at SLAC. The p ositrons are interpreted as arising from a two-step pro cess in which laser photons are backscattered to GeV energies by the electron b eam followed by a collision b etween the high-energy photon and several laser photons to pro duce an electron-p ositron pair. These results are the rst lab oratory evidence for inelastic light-by-light scattering involving only real photons. Submitted to Physical Review Letters Work supp orted by Department of Energy contract DE{AC03{76SF00515 and grants DE{FG02{ 91ER40671, DE{FG02{91ER40685 and DE{FG05{91ER40627. y Present address: Hughes Leitz Optical Technologies Ltd., Midland, Ontario, Canada L4R 2H2. z Present address: Lawrence Livermore National Lab oratory, Livermore, CA 94551. x also Department of Mechanical Engineering { Present address: Panoramastrasse 8, 78589 Durbheim, Germany The pro duction of an electron-p ositron pair in the collision of two real photons was rst considered by Breit and Wheeler [1] who calculated the cross section for the reaction + ! + ! ! e e 1 1 2 2 to b e of order r , where r is the classical electron radius. While pair creation by real photons e e is b elieved to o ccur in astrophysical pro cesses [2] it has not b een observed in the lab oratory up to the present. After the invention of the laser the prosp ect of intense laser b eams led to reconsideration of the Breit-Wheeler pro cess by Reiss [3] and others [4, 5 ]. Of course, for pro duction of an electron-p ositron pair the center-of-mass CM energy of the scattering photons must 2 b e at least 2mc 1 MeV. While this precludes pair creation by a single electromagnetic wave, the necessary CM energy can b e achieved by colliding a laser b eam against a high- energy photon b eam created, for example, by backscattering the laser b eam o a high-energy electron b eam. With laser lightofwavelength 527 nm energy 2.35 eV, a photon of energy 111 GeV would b e required for reaction 1 to pro ceed. However, with an electron b eam of energy 46.6 GeV as available at the Stanford Linear Accelerator Center SLAC the maximum Compton-backscattered photon energy from a 527-nm laser is only 29.2 GeV. In strong electromagnetic elds the interaction need not b e limited to initial states with two photons [3], but rather the number of interacting photons b ecomes large as the dimen- q 2 2 sionless, invariant parameter = e hA A i =mc = eE =m! c = eE =mc approaches rms 0 rms 0 or exceeds unity. Here the laser b eam has lab oratory frequency ! , reduced wavelength , 0 0 ro ot-mean-square electric eld E , and four-vector p otential A ; e and m are the charge rms and mass of the electron, resp ectively, and c is the sp eed of light. For photons of wavelength 527 nm a value of = 1 corresp onds to lab oratory eld 10 19 2 strength of E =610 V/cm and intensity I =10 W/cm . Suchintensities are now lab practical in tabletop laser systems based on chirp ed-pulse ampli cation [6]. Then the multiphoton Breit-Wheeler reaction + ! + n! ! e e 2 0 b ecomes accessible for n 4 laser photons of wavelength 527 nm colliding with a photon of energy 29 GeV. Similarly the trident pro cess 0 + e + n! ! e e e 3 0 requires at least ve 527-nm laser photons colliding with an electron of 46.6 GeV. Reaction + 3 is a variant of the Bethe-Heitler pro cess [7] in whichan e e pair is created by the interaction of a real photon with a virtual photon from the eld of a charged particle. When an electromagnetic eld with 4-tensor F is prob ed by a particle of 4-momentum p an invariant measure of the strength of vacuum-p olarization e ects is q 2 2 3 2 16 2 = hF p i=mc E , where E = m c =eh = mc =e =1:310 V/cm is the crit crit C quantum electro dynamic QED critical eld strength [8, 9 ] at which the energy gain of an electron accelerating over a Compton wavelength is its rest energy, and at whicha C static electric eld would sp ontaneously break down into electron-p ositron pairs. Indeed, 2 the predicted rates [3, 4 , 5] for reaction 2 b ecome large only when approaches unity, and not necessarily when b ecomes large. When a photon of energyh! collides head-on with a wave of lab oratory eld strength 2 2 E and invariant strength , the invariant = 2h ! =mc E =E = 2h ! =mc = rms rms crit C 0 may b e large. For example, in a head-on collision of a photon of energy 29 GeV with a 527-nm laser pulse = 84 nm, =0:52 . 0 Likewise, in reaction 3, or other e-laser interactions involving vacuum p olarization, the ? ? relevantinvariantis=E =E , where E =2 E is the laser eld strength as viewed in crit rms 2 the rest frame of an electron b eam with lab oratory energy E and Lorentz factor = E =mc . For a 46.6-GeV electron b eam colliding head-on with a 527-nm laser, = 0:84 . Wehave p erformed an exp erimental study of strong- eld QED in the collision of a 46.6- GeV electron b eam, the Final Fo cus Test Beam FFTB at SLAC [10], with terawatt pulses from a frequency doubled Nd:glass laser with a rep etition rate of 0.5 Hz achieved by a nal laser ampli er with slab geometry [11, 12 , 13 , 14]. A schematic diagram of the exp eriment is shown in Fig. 1. The apparatus was designed to detect electrons that undergo nonlinear Compton scattering 0 e + n! ! e + ! 4 0 as well as p ositrons pro duced in e-laser interactions. Measurements of reaction 4 have b een rep orted elsewhere [11 , 15]. Figure 1: Schematic layout of the exp eriment. The p eak fo cused laser intensitywas obtained for linearly p olarized green 527 nm pulses 2 of energy U = 650 mJ, fo cal area A 2 =30 m and width t =1:6 ps fwhm, for x y 18 2 which I = U=At 1:3 10 W/cm , =0:36, =0:2 and = 0:3. The electron b eam was op erated at 10-30 Hz and was tuned to a fo cus with =25 m x and =40m at the laser-electron interaction p oint. Typical bunches were 7 ps long y 9 fwhm and contained 7 10 electrons. A string of p ermanent magnets after the collision p oint de ected the electron b eam downwards by 20 mrad. Electrons and p ositrons of momenta less than 20 GeV were de- ected by the magnets into two Si-W calorimeters ECAL and PCAL with energy resolu- q tion =E 19= E [GeV] and p osition resolution of 2 mm. The Si-W calorimeters were E 3 calibrated in parasitic running of the FFTB in which linac-halo electrons of energies b etween 5 and 25 GeV were transmitted by the FFTB when the latter was tuned to a lower energy. Electrons scattered via reaction 4 for n = 1, 2 and 3 laser photons were measured in gas Cerenkov counters lab eled EC37, N2 and N3 in Fig. 1. We used detectors based on Cerenkov radiation b ecause of their insensitivity to ma jor sources of low-energy background. EC37 was calibrated by inserting a thin foil in the electron b eam at IP1. The momentum acceptance and eciency of the counters N2 and N3 were measured with the parasitic electron b eam by comparison with the previously calibrated ECAL. The spatial and temp oral overlap of the electron and laser b eams was optimized by observing the Compton scattering rate in the EC37, N2, N3 and ECAL detectors during horizontal, vertical, and time scans of one b eam across the other. We used the PCAL calorimeter to search for p ositrons pro duced at IP1. Because of the high rate of electrons in the ECAL calorimeter from Compton scattering it was not p ossible to identify the electron partners of the p ositrons. 2 ] clu /p mm [ clu 1.5 100 ratio E 1 50 cluster Ypos 0.5 (a) (b) 0 0 0 50 100 -10 0 10 cluster Ypos [mm] cluster Xpos [mm] Figure 2: Cluster densities from p ositrons pro duced by a wire inserted at IP1.
Details
-
File Typepdf
-
Upload Time-
-
Content LanguagesEnglish
-
Upload UserAnonymous/Not logged-in
-
File Pages9 Page
-
File Size-