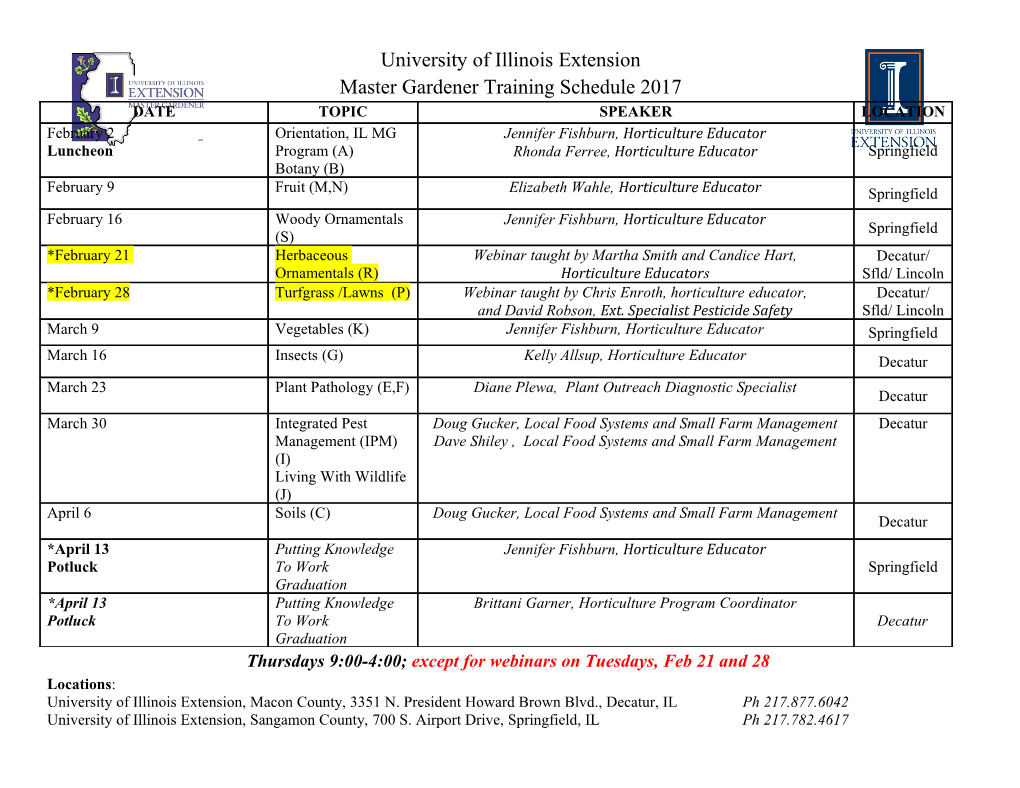
ARTICLE Received 20 Apr 2014 | Accepted 29 Aug 2014 | Published 29 Sep 2014 DOI: 10.1038/ncomms6107 Antarctic contribution to meltwater pulse 1A from reduced Southern Ocean overturning N.R. Golledge1,2, L. Menviel3,4, L. Carter1, C.J. Fogwill3, M.H. England3,4, G. Cortese2 & R.H. Levy2 During the last glacial termination, the upwelling strength of the southern polar limb of the Atlantic Meridional Overturning Circulation varied, changing the ventilation and stratification of the high-latitude Southern Ocean. During the same period, at least two phases of abrupt global sea-level rise—meltwater pulses—took place. Although the timing and magnitude of these events have become better constrained, a causal link between ocean stratification, the meltwater pulses and accelerated ice loss from Antarctica has not been proven. Here we simulate Antarctic ice sheet evolution over the last 25 kyr using a data-constrained ice-sheet model forced by changes in Southern Ocean temperature from an Earth system model. Results reveal several episodes of accelerated ice-sheet recession, the largest being coincident with meltwater pulse 1A. This resulted from reduced Southern Ocean overturning following Heinrich Event 1, when warmer subsurface water thermally eroded grounded marine-based ice and instigated a positive feedback that further accelerated ice-sheet retreat. 1 Antarctic Research Centre, Victoria University of Wellington, Wellington 6140, New Zealand. 2 GNS Science, Avalon, Lower Hutt 5011, New Zealand. 3 Climate Change Research Centre, University of New South Wales, Sydney, New South Wales 2052, Australia. 4 ARC Centre of Excellence for Climate System Science, Sydney, New South Wales 2052, Australia. Correspondence and requests for materials should be addressed to N.R.G. (email: [email protected]). NATURE COMMUNICATIONS | 5:5107 | DOI: 10.1038/ncomms6107 | www.nature.com/naturecommunications 1 & 2014 Macmillan Publishers Limited. All rights reserved. ARTICLE NATURE COMMUNICATIONS | DOI: 10.1038/ncomms6107 eologically constrained numerical model simulations incorporates a high southern latitude freshwater input (freshwater indicate that during the last glacial termination (T1, 19– forcing (FWF)) at the time of MWP1A, and one that does not (no G11 ka before present (BP)) the Antarctic ice sheets (AIS) freshwater forcing (NFW))11 (Supplementary Fig. 1b). As the two contributed 10–18 m to global mean sea level (GMSL) rise1–5. Earth System model simulations differ only in the prescription (or However, whether the AIS made a substantial contribution to not) of a Southern Ocean freshwater flux, we are able to use these deglacial meltwater pulses is still a matter of debate, as a well- two scenarios to isolate the differences in ice-sheet response that constrained quantitative estimate of the volume, timing and rate arise solely as a consequence of this forcing. Other than the of freshwater flux from the AIS is still lacking. For Antarctica to different sea level and ocean heat forcings, all of the deglacial have made a meaningful contribution to deglacial meltwater simulations use identical glaciological parameterization, time- pulses (MWP1A and MWP1B) during T1 (hereafter referred to as dependent air temperature and precipitation forcings, as well as the ‘AIS MWP theory’), three conditions must be satisfied. First, an isostatic model. Based on the ensemble mean, the simulations sufficient sea-level equivalent (s.l.e.) ice volume must have existed reveal that centennial-scale periods of accelerated ice mass loss in Antarctica at the Last Glacial Maximum (LGM). Second, this could have occurred during the last glacial termination, with the ice volume must have been discharged at the correct time and at a largest such event coinciding with MWP1A. However, for the AIS fast-enough rate to contribute to the rapid rises in sea level. Third, to have contributed substantially to this event we find that a a plausible mechanism must exist by which the previous two specific interaction between the ice sheet and its surrounding ocean conditions are met. is necessary. Specifically, mass loss rates are highest when an Here we present a suite of ice-sheet modelling experiments that abrupt reduction in Southern Ocean overturning allows rapid employ a range of proxy-based and modelled sea level and ocean subsurface oceanic warming to take place. temperature time series to generate a suite of possible Antarctic ice-sheet retreat scenarios. Our simulations use the latest sea-level reconstruction from Tahiti6, the most probable (centreline) Results scenario from a probabilistic assessment of six far-field sea-level Fit to LGM ice extent. To produce an ice-sheet geometry that records7, and from a glacio-isostatic adjustment model simulation closely agrees with ice-core interpretations of LGM surface of eustatic sea level8 (Supplementary Fig. 1a). We combine each of elevation changes and marine geological reconstructions of LGM these with each of four scenarios representing changes in ocean extent, and reproduces the pattern of temporal evolution in each heat through T1, from a global stack of 57 deep-ocean records9, sector of the ice sheet, we ran a suite of simulations representing a a Southern Ocean-specific record from offshore New Zealand10 range of glaciological parameterizations. Outputs from each and from two transient simulations of the last deglaciation experiment were assessed against a range of empirical constraints using an intermediate complexity Earth System model—one that to gauge overall closeness-of-fit (see Methods). The simulation 2,900 4,000 2,800 −120 m (−138 m) 3,900 2,700 Dome F 3,800 2,600 +17 m (10 m) Epica DML 3,700 EPICA DML −100 − +60 m 2,500 3,600 2,400 Dome F 3,500 −120 m 1,200 3,700 1,100 −120 m (−151 m) 3,600 1,000 +478 m (859 m) Vostok 3,500 900 Berkner island Berkner Isl. 3,400 +1,400 m 800 East 3,300 700 Antarctica 3,200 600 WAIS divide +200 m Vostok 3,300 Byrd station −100 m 3,200 1,900 +200 m +260 m (376 m) Dome C 3,100 1,800 WAIS divide Siple dome +200 − +400 m −120 m −176 m (−220 m) 3,000 1,700 Roosevelt Isl. 2,900 Elevation (m) 1,600 unkn. Taylor dome Dome C < +50 m Law dome 2,800 +136−335 m 2,000 Talos dome 1,900 unkn. 1,500 1,800 +96 m (116 m) 1,400 1,700 +155 m (241 m) 10−1 100 101 102 103 Law dome 1,300 Byrd station 1,600 Surf. vel (ma−1) 1,200 1,500 1,100 1,000 1,200 900 +501 m (664 m) +458 m (676 m) 1,100 800 2,600 2,400 Siple dome Roosevelt island 1,000 700 +0 m (102 m) 2,500 −108 m (−89 m) 2,300 900 600 2,400 Talos dome 2,200 800 500 2,300 2,100 Taylor dome 700 400 2,200 2,000 600 300 2,100 1,900 25 20 15 10 5 0 25 20 15 10 5 0 25 20 15 10 5 0 25 20 15 10 5 0 Model years (ka BP) Model years (ka BP) Model years (ka BP) Model years (ka BP) Figure 1 | Geometry and dynamics of the ensemble mean LGM AIS. Main panel shows locations of ice cores used for constraint and their interpreted LGM elevation or thickness change from present. Geologically interpreted LGM grounding-line positions shown with dashed line18. Marginal panels show time series of modelled surface elevation changes at each of the ice core sites. Simulated LGM to present-day elevation change and thickness at each ice core site are shown in bold and parenthesis respectively. ‘LGM’ in this case is defined as the maximum elevation/thickness reached during the period 25–20 ka BP, thus may not be contemporaneous between sites. Red squares denote present-day ice-sheet surface elevations. 2 NATURE COMMUNICATIONS | 5:5107 | DOI: 10.1038/ncomms6107 | www.nature.com/naturecommunications & 2014 Macmillan Publishers Limited. All rights reserved. NATURE COMMUNICATIONS | DOI: 10.1038/ncomms6107 ARTICLE considered to reflect the ‘best-fit’ to these constraints is shown in not only that the glaciological parameterization is well-tuned, but Fig. 1, based on the parameterization described in Supplementary also that the transient forcings are appropriately scaled. Table 1 and the ice-core constraints listed in Supplementary For comparison with measured bedrock uplift rates around the Table 2. The pattern of LGM thickening with respect to modelled continent, we extract the pattern and magnitude of the ongoing present-day ice thickness is robustly captured at most sites, rebound signal from the final time slice of our model. Residual although the model underestimates absolute present-day eleva- uplift maxima lie in the inner Weddell and Ross seas, with lower tions at Law Dome by B18%. In general, mismatches in modelled rates around most of the modern coastline and across most of the versus observed surface elevation at the end of the model run are Antarctic Peninsula. Bedrock elevation change is close to zero for most pronounced on coastal islands, such as Law Dome, interior East Antarctica and central WAIS, with slow subsidence Berkner Island and Roosevelt Island. Model-data fit is far modelled in some sectors (Supplementary Fig. 3). Despite better in continental interior areas such as Dome F (o1% dif- considerable uncertainties in the Global Positioning System ference in elevation), Vostok (o3%), Dome C (o1%) and the (GPS)-derived data, the overall pattern shown by the model is West Antarctic Ice Sheet (WAIS) Divide (o6%). This gives us consistent with the pattern indicated by the available measure- confidence that the ice-sheet model is performing reliably, and we ments (Supplementary Fig. 3). attribute the peripheral mismatches primarily to unintended consequences of the spin-up procedure, principally the initial smoothing run that relaxes the ice surface from its initial state to Evolution of ice-sheet geometry.
Details
-
File Typepdf
-
Upload Time-
-
Content LanguagesEnglish
-
Upload UserAnonymous/Not logged-in
-
File Pages10 Page
-
File Size-