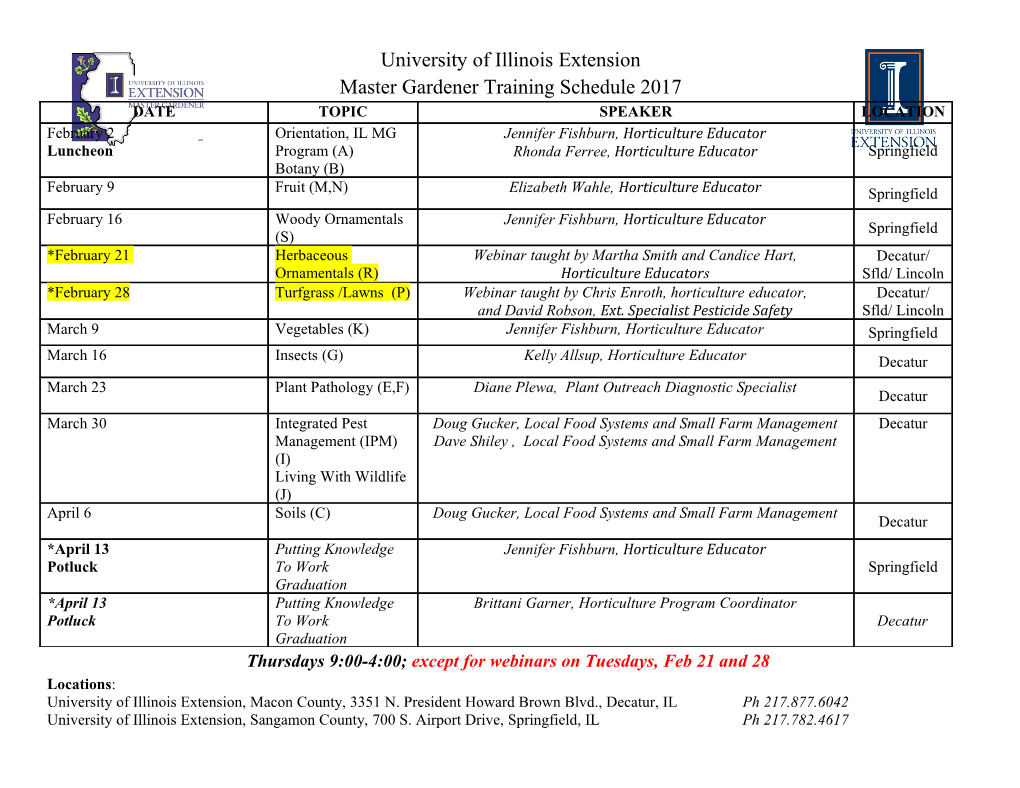
Growing Urban Bicycle Networks Michael Szell∗a,b, Sayat Mimarc, Tyler Perlmanc, Gourab Ghoshalc, and Roberta Sinatraa,b aNEtwoRks, Data, and Society (NERDS), IT University of Copenhagen, 2300 Copenhagen, Denmark bComplexity Science Hub Vienna, 1080 Vienna, Austria cDepartment of Physics and Astronomy, University of Rochester, Rochester, NY 14627, USA Abstract Cycling is a promising solution to unsustainable car-centric urban transport systems. However, prevailing bicycle network development follows a slow and piecewise process, without taking into account the structural complexity of transportation networks. Here we explore systematically the topological limitations of urban bicycle network development. For 62 cities we study different variations of growing a synthetic bicycle network between an arbitrary set of points routed on the urban street network. We find initially decreasing returns on investment until a critical threshold, posing fundamental consequences to sustainable urban planning: Cities must invest into bicycle networks with the right growth strategy, and persistently, to surpass a critical mass. We also find pronounced overlaps of synthetically grown networks in cities with well-developed existing bicycle net- works, showing that our model reflects reality. Growing networks from scratch makes our approach a generally applicable starting point for sustainable urban bicycle network planning with minimal data requirements. Introduction tion, and climate, reveals that in the EU alone cy- cling brings a yearly benefit worth e 24 billion while Cities worldwide are scrambling for sustainable solu- automobility costs society e 500 billion [4]. These in- tions to their inefficient, car-centric transport systems sights provide further impetus for coordinated efforts [1,2]. One promising, time-tested candidate is cycling. to extend cycling infrastructure as one solution to the It is an efficient mode of sustainable urban transport urban transport crisis and to effectively fight climate that can account for the majority of intra-urban trips change [5, 6]. which are primarily short and medium-distance [3]. In practice, however, bicycle infrastructure devel- Cost-benefit analysis that accounts for health, pollu- opment struggles with a particularly pervasive polit- *Corresponding author. Email: [email protected] ical inertia due to “car culture” [7, 8]: For example, Copenhagen took 100 years of political struggles to develop a functioning grid of protected on-street bi- a cycle networks [9] that continues to be split into 300 disconnected components today [10]. Accordingly, the most developed, influential bicycle network planning guidelines, such as the Dutch CROW manual [11], ac- knowledge that building up bicycle networks happens typically through decades-long, piecewise refinements. Unfortunately, given the advanced stage of the plane- b c tary climate crisis [12], humanity has run out of time – there is now no other choice left than making ur- ban transport sustainable as fast as possible [1, 7, 13]. While there has been historical inertia, the ongoing arXiv:2107.02185v2 [physics.soc-ph] 13 Jul 2021 COVID-19 pandemic has prompted several cities to engage in successful accelerated network development, proving that such efforts are indeed possible [14, 15]. A systematic exploration of city-wide, comprehensive development strategies is therefore urgently needed. Figure 1: The state of existing bicycle networks. a We extract street networks from 62 cities covering differ- Although the prevailing, piecewise application of ent regions and cultures; many are considered modern and qualitative policy guidelines in existing bicycle network well developed. b The distribution of city-wide lengths of planning [11, 16] might have a good track record in bicycle tracks indicates negligible existing cycling infras- e.g. Dutch cities and Copenhagen [9], this process lacks tructure that is also c split into hundreds of disconnected rigorous scrutiny: Are the resulting networks truly op- components. See more details in Supplementary Table 1. timal? Can such policies be replicated in other cities? 1 And are there fundamental topological limitations for Minimum spanning tree Triangulation Fully connected developing a bicycle network? Indeed, an evidence- based, scientific theory of bicycle network development is missing. There is a growing academic literature on analyzing Investor's Cohesive Traveler's bicycle networks of specific cities, for instance Mon- optimum planar network optimum treal [17], Seattle [18], or recent data-driven approaches Resilient for Bogota [19] or London [20]. While such stud- Economic ies are invaluable in terms of local enhancements and Figure 2: Optimal connected network solutions. data consolidation for a particular place, here we in- Adapted from Ref. [29]. (Left) The investor’s optimal strat- stead focus on a global analysis, in particular on the egy for a connected network is to invest as little as possible, fundamental topological limitations of bicycle network minimizing total link length [10]. Its solution is a minimum development that are relevant for all urban environ- spanning tree, maximally economic but minimally resilient ments, independent of the availability of traffic flow with low directness, inadequate for travelers. (Right) The data [21]. This approach follows the idea of a Science traveler’s optimum connects all node pairs creating all di- of Cities [22] where we study the topological proper- rect routes. This solution is minimally economic, maxi- ties of bicycle networks that are independent of place mally resilient and direct, inadequate for investors. It also has crossing links and is therefore not a planar network. using computational, quantitative methods of Urban (Center) A both economic and resilient, as well as cohe- Data Science [23]. sive planar network solution in-between is the triangulation. In particular the minimum weight triangulation, approxi- The vast majority of cities on the planet has neg- mated by the greedy triangulation, minimizes investment. ligible infrastructure for safe cycling [6]. Indeed, ur- ban transport infrastructure development worldwide has been heavily skewed towards automobiles since Results the 20th century, today featuring well connected net- works of streets for motorized vehicles [10]. Rather The starting point for our analysis is the street net- than uprooting the existing infrastructure and replac- works of 62 cities, see Fig. 1(a) and Supplementary ing it with an entirely new one—an economically infea- Table 1. Here, links represent streets and nodes are sible strategy—we investigate how to retrofit existing street intersections. Being embedded in a metric space, streets into bicycle networks. Sacrificing specificity for these constitute planar graphs [30]. We downloaded generalizability, our formulation contains as a starting and processed these networks from OpenStreetMap us- point two ingredients: the existing street network of a ing OSMnx [31] (see Methods). city, and an arbitrary set of points of interests. With Although many of the covered cities are from well these minimal ingredients we explore different growth developed regions, we observe that they have negli- strategies that sequentially convert streets that were gible bicycle infrastructure, Fig. 1(b). Additionally designed for only cars to streets that are safe for cy- these are split into hundreds of disconnected compo- cling [11, 24]. Inspired by the CROW manual [11], the nents, Fig. 1(c), which has previously prompted anal- objective of all explored strategies is to create cohe- ysis of strategies to merge them [10]. Although such sive networks, i.e. well connected networks that cover strategies make sense in cities with already well es- a large fraction of the city area (see Methods). tablished bicycle infrastructure, they are less useful in most other cities. Further, they produce minimum Across the realistic strategies we report a growth spanning tree-like solutions that are economically at- phase that initially leads to a diminishing trend of tractive but lack resilience and cohesion (Fig. 2), and quality indicators, until a critical fraction of streets are they potentially reinforce socioeconomic inequalities by converted, akin to a percolation transition observed in connecting only already developed areas while ignoring critical phenomena and also present in the growth of under-developed ones [21], prompting us here to grow other forms of transportation infrastructure as well as new networks from scratch instead. patterns of traffic [19, 21, 25–28]. In other words, ini- tial investments into building cycling-friendly infras- Growing bicycle networks from scratch tructure leads to diminishing returns on quality and efficiency until the emergence of a well-connected giant Our process of growing synthetic bicycle networks con- component. Once this threshold is reached, the quality sists of four steps, Fig. 3, starting with the street improves dramatically, to an extent which depends on network and the points of interest. For details see the specific growth strategy. We provide empirical evi- Methods; for an intuitive, interactive exploration see dence that the majority of cities effectively lie below the http://growbike.net. threshold which might be hindering further growth, im- plying fundamental consequences to sustainable urban Step 1) Points of interest (POIs). An arbitrary planning policy: To be successful, cities must invest set of POIs is snapped to the intersections of the street into bicycle networks
Details
-
File Typepdf
-
Upload Time-
-
Content LanguagesEnglish
-
Upload UserAnonymous/Not logged-in
-
File Pages26 Page
-
File Size-