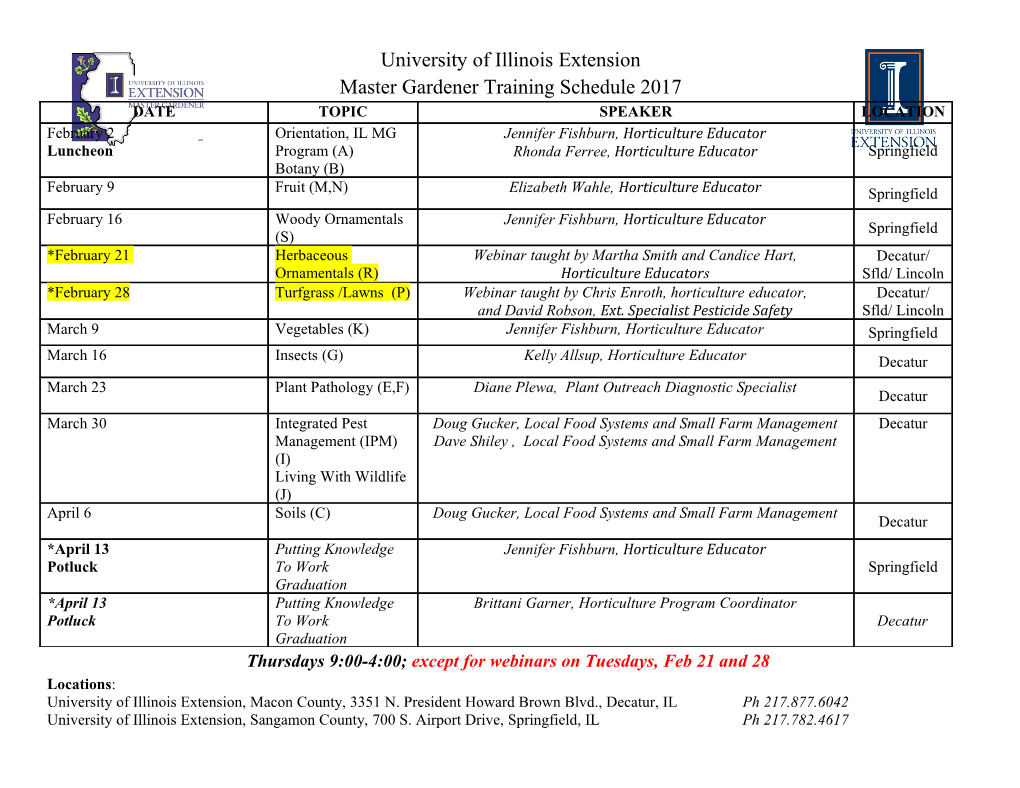
Gravitational and Aharonov-Bohm Phases in Neutron Interferometry Dissertation zur Erlangung des Grades eines Doktors der Naturwissenschaften eingereicht von Gerbrand van der Zouw, M.Sc. durchgef¨uhrtam Institut f¨urExperimentalphysik der Universit¨atWien bei o. Univ. Prof. Dr. Anton Zeilinger Diese Arbeit wurde durch den Fond zur F¨orderungwissenschaftlicher Forschung (Projekt S6503) und durch die Europ¨aische Union (TMR Network No. ERB-FMRX-CT96-0057) unterst¨utzt. Copyright c 2000 by G. van der Zouw All rights reserved. No part of this publication may be reproduced, stored in a retrieval system or transmitted in any form or by any means, electronic, mechanical, photocopying, recording or otherwise, without the prior written permission of the author. v Abstract This thesis describes two experiments with the interferometer for very-cold-neutrons (VCN) at the Institut Laue-Langevin in Grenoble, France. This interferometer operates with neu- trons that are 40 m/s slow and uses micro-fabricated phase gratings as beam-splitters. The first experiment is a demonstration of the scalar neutron Aharonov-Bohm (AB) effect, which is the neutron analogue of the electrostatic AB effect for electrons. Aharonov and Bohm (1959) proposed this latter effect together with the magnetic AB effect to clar- ify the significance of electromagnetic potentials in quantum mechanics. The experiment described here focuses at for the first time simultaneously demonstrating the two essen- tial operational signatures of all AB-type effects: their nondispersivity, i.e. wavelength independence, and the fact that they are are not locally observable in a simply connected space-time. In this thesis it will be shown, as was already pointed out by Zeilinger (1984, 1986), that the neutron analogue also has both signatures and thus can be used to demon- strate them. In the experiment the paths of the neutron interferometer are enclosed by two anti- parallel solenoids. When, determined by a chopper, the neutron is completely inside the homogeneous field region of the coils the field is turned on and off, resulting in a phase shift due to the difference in magnetic energy. High-visibility fringes were observed even for phase shifts exceeding the limit given by the coherence length, which is a clear demon- stration of the wavelength independence of this effect. Because the interferometer has spatially separated beams and uses unpolarized neutrons the effect of the magnetic field on the neutron is not locally observable in either of the interferometer arms. The second experiment is what is commonly known as a COW-experiment. In these experiments a neutron interferometer is tilted around its optical axis, resulting in a phase shift due to the difference in gravitational potential acting along the different beam paths. It is a long-standing issue in the field of neutron interferometry, that a whole series of experiments using silicon single-crystal interferometers, starting with the original experi- ment by Colella, Overhauser, and Werner (1975), consistently show a discrepancy between experiment and theory, after correction for dynamical diffraction effects and other known systematic errors. This situation prompted the measurement of the COW phase shift with the VCN interferometer, which, because of its different characteristics, is not expected to suffer from the same systematic problems. Weber (1998) obtained results that were in agreement with theory within the accuracy of 1%, excluding some, but not all of the earlier results. By tailoring the incoming neutron spectrum and by more thoroughly investigating and eliminating the systematic effects, the new measurements described in this thesis have a significantly higher accuracy of 0.4%, confirming theory while excluding at the same time all earlier anamolous results on a 1σ-level. vi Abstract/Kurzfassung vii Kurzfassung In der vorliegenden Dissertation werden zwei Experimente mit dem Interferometer sehr kalter Neutronen am Institut Laue-Langevin in Grenoble, Frankreich beschrieben. Dieses Interferometer verwendet Neutronen, die nur 40 m/s schnell sind. Als Strahlteiler werden mikro-fabrizierte Phasengitter eingesetzt. Das erste Experiment ist eine Demonstration des skalaren Aharonov-Bohm (AB) Ef- fektes fur¨ Neutronen. Dieser Effekt ist das Neutronenanalogon des elektrostatischen AB Effektes fur¨ Elektronen. Aharonov und Bohm (1959) haben diesen Effekt zusammen mit dem magnetischen AB Effekt vorgeschlagen zur Erkl¨arung der Signifikanz elektromagneti- scher Potentiale in der Quantenmechanik. Der hier beschriebene Versuch konzentriert sich vor allem auf die erstmalig gleichzeitige Demonstration der zwei essenziellen operationellen Merkmale aller AB-¨ahnlichen Effekte: ihre Nichtdispersivit¨at, d.h. ihre Wellenl¨angenun- abh¨angigkeit, und die Tatsache, daß sie in einer einfachzusammenh¨angenden Raumzeit nicht lokal beobachtbar sind. In dieser Dissertation wird, wie schon vorher von Zeilinger (1984, 1986), gezeigt, daß das Neutronenanalogon diese Merkmale auch besitzt und daher zu ihrer Demonstration genutzt werden kann. Bei dem Versuch werden die Pfade des Neutroneninterferometers von zwei entgegen- gerichteten Solenoiden umgeben. Wenn, von einem Chopper bestimmt, das Neutron sich vollkommen innerhalb des homogenen Feldbereiches der Spulen befindet, wird das Feld an- und abgeschaltet, was zu einem von der magnetischen Energiedifferenz gegebenen Phasenschub fuhrt.¨ Sogar fur¨ Phasenschube¨ weit jenseits des von der Koh¨arenzl¨ange vorgegebenen Limes wurden noch hochaufgel¨oste Interferenzstreifen beobachtet, was eine eindeutige Demonstration der Nichtdispersivit¨at darstellt. Weil das Interferometer r¨aum- lich getrennte Strahle hat und unpolarisierte Neutronen verwendet werden, ist der Einfluß des Magnetfeldes auf das Neutron in keinem der Interferometerpfade lokal beobachtbar. Der zweite Versuch ist in der Literatur als COW-Experiment bekannt. Bei dieser Art von Experimenten wird das Neutroneninterferometer um seine optische Achse gedreht, was einen Phasenschub zufolge hat, der von der Differenz des Schwerkraftspotentials zwischen den beiden Interferometerwegen bestimmt ist. Eine lang ungekl¨arte Frage der Neutro- neninterferometrie besteht darin, daß in den bisherigen Experimenten mit Siliziumper- fektkristallinterferometern schon seit dem ersten Experiment durch Collela, Overhauser und Werner (1975) eine Diskrepanz zwischen Experiment und theoretischer Vorhersage beobachtet wird, selbst nach Korrekturen fur¨ dynamische Beugung und andere, bekannte systematische Fehler. Diese Situation war der Anlaß dafur,¨ eine Messung des COW-Phasenschubes mit dem Interferometer sehr kalter Neutronen durchzufuhren,¨ da dieses Interferometer wegen sei- ner verschiedenen Eigenschaften nicht den gleichen systematischen Effekten ausgesetzt viii Abstract/Kurzfassung ist. Die Ergebnisse von Weber (1998) stimmen innerhalb einer Genauigkeit von 1% mit der Theorie uberein,¨ schließen dennoch nicht alle von der Theorie abweichenden fruheren¨ Ergebnisse aus. W¨ahrend dieser Doktorarbeit wurde das Neutronenspektrum besser der Messung angepaßt und die systematischen Effekte weiter untersucht und eliminiert. Die neuen Meßergebnisse mit einer wesentlich besseren Genauigkeit von 0.4% best¨atigen die theoretische Vorhersage und schließen alle fruheren¨ abweichenden Ergebnisse innerhalb der Standardabweichung aus. ix Contents Abstract/Kurzfassung v 1. Introduction 1 2. Neutron optics and interferometry 3 2.1. The neutron . 3 2.2. Neutron optics . 3 2.2.1. The analogy with geometrical optics . 3 2.2.2. Interaction between neutrons and matter . 5 2.2.3. Reflection . 8 2.3. Neutron interferometry . 10 2.3.1. Calculation of interference phases . 11 2.3.2. Experimental considerations . 16 2.4. Overview of different types of neutron interferometers . 17 2.4.1. Perfect crystal interferometers . 19 2.4.2. Grating interferometers . 20 3. The very-cold-neutron interferometer 27 3.1. Introduction . 27 3.2. Components of the VCN interferometer . 27 3.2.1. Support and housing . 27 3.2.2. Incoming neutron beam . 28 3.2.3. The neutron interferometer . 30 3.2.4. Detection . 32 3.2.5. Electronics and software . 32 3.3. Contrast . 33 3.3.1. Tilt of the gratings . 33 3.3.2. Undesired diffraction orders . 36 3.3.3. Background . 36 3.4. Stability . 37 3.4.1. Temperature stabilisation . 38 3.4.2. Stabilisation of the interferometer tilt . 39 4. Nondispersivity of the Aharonov-Bohm effect 43 4.1. Introduction . 43 4.2. Overview of Aharonov-Bohm type effects . 43 4.2.1. The original Aharonov-Bohm effects . 43 x Contents 4.2.2. The Aharonov-Casher effect . 45 4.2.3. The He-McKellar-Wilkens and the dual AB effect . 45 4.3. Aharonov-Bohm effects and the framework of quantum mechanics . 46 4.3.1. Topology of the Aharonov-Bohm effects . 46 4.3.2. Potentials and the Aharonov-Bohm effect . 47 4.3.3. Bohr's complementarity principle . 48 4.3.4. Nonlocality . 49 4.3.5. Operational signatures of Aharonov-Bohm phase shifts . 50 4.4. Scalar neutron AB (SNAB) effect . 51 4.4.1. Analysis of SNAB effect using spinors . 51 4.4.2. Analysis of the SNAB effect using density matrices . 53 4.4.3. Phase shift from a static magnetic field . 55 4.5. Earlier experiments . 56 4.6. Design of the experiment . 57 4.6.1. Overall design requirements . 58 4.6.2. Coil . 59 4.6.3. Chopper . 62 4.6.4. Timing of chopper and field pulse . 64 4.6.5. Electronics . 65 4.7. Experimental results . 70 4.7.1. Preparatory measurements . 70 4.7.2. Phase shift from a static magnetic field . 72 4.7.3. Measurement of the SNAB-phase shift . 75 4.8. Conclusions . 77 5.
Details
-
File Typepdf
-
Upload Time-
-
Content LanguagesEnglish
-
Upload UserAnonymous/Not logged-in
-
File Pages180 Page
-
File Size-