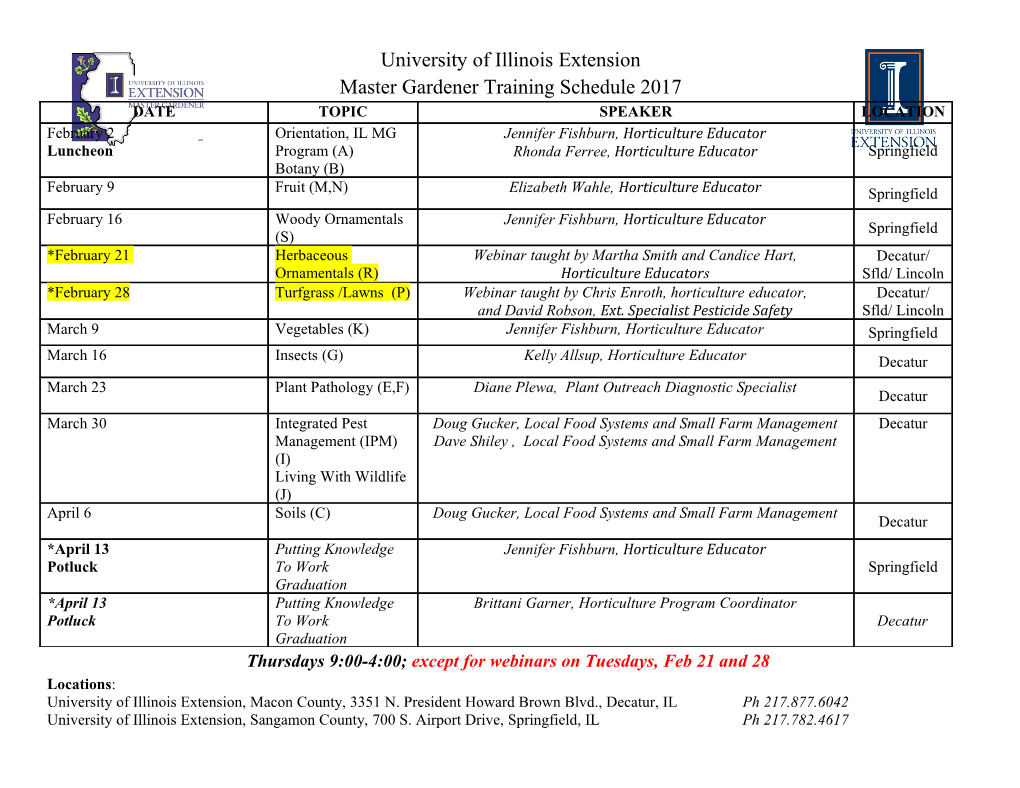
Shared dynamics of LeuT superfamily members and allosteric differentiation rstb.royalsocietypublishing.org by structural irregularities and multimerization Research Luca Ponzoni†, She Zhang†, Mary Hongying Cheng† and Ivet Bahar Cite this article: Ponzoni L, Zhang S, Cheng Department of Computational and Systems Biology, School of Medicine, University of Pittsburgh, Pittsburgh, PA 15213, USA MH, Bahar I. 2018 Shared dynamics of LeuT superfamily members and allosteric LP, 0000-0001-8125-582X; SZ, 0000-0001-9265-4498; MHC, 0000-0001-5833-8221; IB, 0000-0001-9959-4176 differentiation by structural irregularities and multimerization. Phil. Trans. R. Soc. B 373: The LeuT-fold superfamily includes secondary active transporters from 20170177. different functional families, which share a common tertiary structure, despite http://dx.doi.org/10.1098/rstb.2017.0177 having a remarkably low sequence similarity. By identifying the common structural and dynamical features upon principal component analysis of a comprehensive ensemble of 90 experimentally resolved structures and an- Accepted: 19 December 2017 isotropic network model evaluation of collective motions, we provide a unified point of view for understanding the reasons why this particular fold One contribution of 17 to a discussion meeting has been selected by evolution to accomplish such a broad spectrum of func- issue ‘Allostery and molecular machines’. tions. The parallel identification of conserved sequence features, localized at specific sites of transmembrane helices, sheds light on the role of broken helices (TM1 and TM6 in LeuT) in promoting ion/substrate binding and allosteric Subject Areas: interconversion between the outward- and inward-facing conformations of biophysics, computational biology, structural transporters. Finally, the determination of the dynamics landscape for the biology structural ensemble provides a promising framework for the classification of transporters based on their dynamics, and the characterization of the collective movements that favour multimerization. Keywords: This article is part of a discussion meeting issue ‘Allostery and molecular LeuT-fold, secondary transporters, machines’. elastic network models, intrinsic dynamics, alternating access, conformational changes Author for correspondence: 1. Introduction Ivet Bahar Secondary active transporters translocate small molecules such as neurotransmit- e-mail: [email protected] ters, nutrients and metabolites across cellular membranes, using the energy provided by the co-transport (symport) or exchange (antiport) of ions or other solutes down their electrochemical gradients. Remarkably, several secondary active transporters, though belonging to genetically and functionally distant families, share a common architecture (or fold). Four common folds among trans- porters are the LeuT-, MFS-, GltPh- and NhaA-folds [1,2]. Prototypical proteins, first-resolved in each case, are: the bacterial (Aquifex aeolicus) leucine transporter (figure 1a), a member of the family of neurotransmitter : sodium symporters (NSSs) [5]; a human glucose transporter belonging to the major facilitator super- family [6]; the archaeal aspartate transporter, GltPh,fromPyrococcus horikoshii [7], which has broadly served as a structural model for human excitatory amino acid transporters; and the Naþ/Hþ antiporter, NhaA, from Escherichia coli [8]. An immediate question concerning the selection of a small pool of folds by a †These authors contributed equally to this large number of transporters involved in different functions, and vastly differing study. in their sequence, is what is special about those folds that lend themselves to different functionalities. What are their structural and dynamic characteristics that are exploited, or how do they adapt to different functions? Differences can Electronic supplementary material is available be at various levels, from sequence, to structural motifs, or quaternary online at https://dx.doi.org/10.6084/m9. figshare.c.4050776. & 2018 The Authors. Published by the Royal Society under the terms of the Creative Commons Attribution License http://creativecommons.org/licenses/by/4.0/, which permits unrestricted use, provided the original author and source are credited. EC 2 a c ( )() LeuT AdiC vSGLT rstb.royalsocietypublishing.org DAT BetP CaiT IC Phil. Trans. R. Soc. B (b) Å 6 LeuT 5 4 MhsT DAT 3 Mhp1 2 373 BetP vSGLT 1 : 20170177 AdiC CaiT 0 Figure 1. LeuT-fold shared by monomeric, dimeric and multimeric transporters. (a) LeuT-fold. Selected helices are colour-coded and labelled, using conventional numbering [3]. (b) RMSDs between structurally resolved LeuT-fold monomers/protomers (see electronic supplementary material, table S1). (c) Different oligomer- ization states of LeuT-fold protomers, illustrated for LeuT dimer (PDB: 2A65), AdiC dimer (PDB: 3L1L), vSGLT dimer (PDB: 3DH4), enhanced by interfacial salt-bridge K449-D506, hDAT dimer model [4] and two trimeric forms, BetP (PDB: 4C7R) and CaiT (PDB: 4M8J). Side views shown in all panels, except for the last where top view of CaiT structurally aligned against BetP is shown. Broken helices TM1 and TM6 are coloured in light blue and green; substrate and sodium ions are in cyan and yellow van der Waals (vdW) spheres, respectively. A number of functional residues are shown in vdW spheres: Gly (green) at the substrate-binding site, aromatic EC gating residues (purple), and positively (blue) or negatively (light red) charged residues participating in substrate binding or IC salt-bridge formation. Anionic lipids bound to the BetP trimer are shown in cyan thick-stick representation, and phosphorus atoms, as tan spheres. H7 helices forming the trimeric interface are shown in BetP (orange cylinders) and CaiT (cyan cylinders), along with the interfacial salt-bridge R299-D288. See also figure 2, and the superposition of the transporters in the OFS and IFS in electronic supplementary material, figure S1. organization (for multimeric transporters) while maintaining ‘thin’ (EC) gate, the repacking of transmembrane (TM) helices the tertiary fold. We will focus here on the LeuT-fold superfam- and the opening of the ‘thick gate’ [12], leading to the release of ily, which probably has the broadest representation in the substrate to the other side [24,25]; and the hydration of the Protein Data Bank (PDB) among the four folds. LeuT has inward-facing (IF) vestibule due to the migration of a co- served as a model for exploring the mechanism of action of transported Naþ ion (Na2) has been observed in silico monoamine transporters such as the dopamine transporter [25–29] and suggested experimentally [30] to cooperatively (DAT) [9] or the serotonin transporter (SERT). stabilize LeuT IFS. Coupled ion binding and structural transi- A classical model for the transport mechanism of second- tions have been reported for GltPh [31]; and those between ary transporters is the alternating access model [10,11]: EC/IC gates and TM helical configuration have been observed mainly, the transporter undergoes a structural change from for a glucose transporter belonging to the SWEET family [32]. the outward-facing state (OFS) for substrate/ion uptake from Such couplings suggest an allosteric regulation of transport the extracellular (EC) medium, to the inward-facing state activity [2,33,34], although a systematic quantitative analysis (IFS) for release of its cargo to the intracellular (IC) medium, of collective dynamics has not been carried out across all (or and vice versa to resume the transport cycle. While this model representative) members of a given fold family. has helped appreciate the mechanistic aspects of substrate Another interesting observation is that structurally hom- transport, recent structural data integrated with biochemical ologous transporters function in various oligomerization and computational studies have improved our understanding states, as illustrated in figure 1c for a few members of the of the complex machinery of transporters [1,2,12–15]. These LeuT-fold family. Their protomers retain their fold (and studies led to the definition of rocking bundle for the LeuT- access to OFS and IFS) (electronic supplementary material, fold [16] or rock-switch mechanism for the MFS-fold [17]. In figure S1), suggesting that this modular fold is used to perform the case of glutamate transporters, an elevator-like sliding of transport functions regardless of the oligomerization state. þ the transport domains [18,19] emerged, shared with Na / A similar observation was made in the trimeric GltPh: the proton antiporters or other Naþ/dicarboxylate co-transporters individual protomers exhibit different levels of exposure to [20]. In addition to these global motions, local EC/IC gating either the EC or IC region, without interference from the events, often enabled by the side chain isomerization or reor- trimerization scaffold [35]. Recent examination of DAT dyna- ientation of selected amino acids or small motifs such as mics also suggests that the dimeric architecture may facilitate helical hairpins, have been elucidated. the OFS $ IFS transition [4,36]. Observations in other families More importantly, couplings between global and local also suggest that multimerization can modulate function: e.g. events [2,21] or even the protonation state of residues [22,23] dimerization reduces the substrate-binding affinity of a nitrate have been reported to direct substrate translocation. In LeuT, transporter (MFS-fold) [37]; and a trimeric SWEET transporter binding of substrate from one
Details
-
File Typepdf
-
Upload Time-
-
Content LanguagesEnglish
-
Upload UserAnonymous/Not logged-in
-
File Pages10 Page
-
File Size-