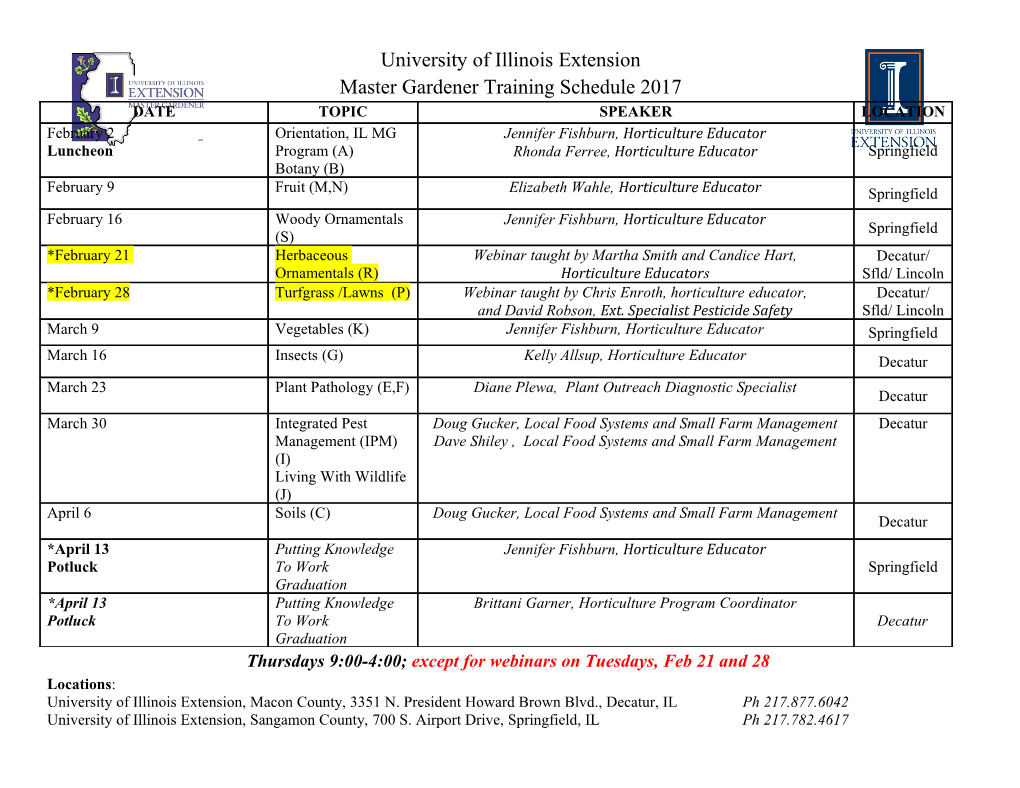
Eur. Phys. J. C (2021) 81:176 https://doi.org/10.1140/epjc/s10052-021-08912-4 Regular Article - Experimental Physics Final results of the LOPES radio interferometer for cosmic-ray air showers LOPES Collaboration W. D. Apel1, J. C. Arteaga-Velázquez2, L. Bähren3, K. Bekk1, M. Bertaina4 , P. L. Biermann5 ,J.Blümer1,6, H. Bozdog1, E. Cantoni4,8 , A. Chiavassa4 , K. Daumiller1, V. de Souza9 , F. Di Pierro4 ,P.Doll1, R. Engel1,6, H. Falcke3,5,10 , B. Fuchs6, H. Gemmeke12, C. Grupen13 , A. Haungs1 , D. Heck1, J. R. Hörandel10 , A. Horneffer5, D. Huber6, T. Huege1 , P. G. Isar14 , K.-H. Kampert11 , D. Kang1 ,O.Krömer12, J. Kuijpers10,K.Link1,a , P. Łuczak15,M.Ludwig6, H. J. Mathes1, M. Melissas6, C. Morello8 , S. Nehls16, J. Oehlschläger1, N. Palmieri6,T.Pierog1 , J. Rautenberg11, H. Rebel1,M.Roth1 , C. Rühle12, A. Saftoiu7 , H. Schieler1, A. Schmidt12, S. Schoo1, F.G. Schröder1,17,b ,O.Sima7,18,G.Toma7,19, G. C. Trinchero8 , A. Weindl1, J. Wochele1 , J. Zabierowski15 , J. A. Zensus5 1 Institute for Astroparticle Physics (IAP) [formerly, Institute for Nuclear Physics (IKP)], Karlsruhe Institute of Technology (KIT), Karlsruhe, Germany 2 Instituto de Física y Matemáticas, Universidad Michoacana, Morelia, Michoacán, Mexico 3 ASTRON, Dwingeloo, The Netherlands 4 Dipartimento di Fisica, Università degli Studi di Torino, Turin, Italy 5 Max-Planck-Institut für Radioastronomie, Bonn, Germany 6 Institute for Experimental Particle Physics (ETP), Karlsruhe Institute of Technology (KIT), Karlsruhe, Germany 7 National Institute of Physics and Nuclear Engineering, Bucharest-Magurele, Romania 8 Osservatorio Astrofisico di Torino, INAF Torino, Turin, Italy 9 Instituto de Física de São Carlos, Universidade de São Paulo, São Carlos, Brasil 10 Department of Astrophysics, Radboud University Nijmegen, AJ Nijmegen, The Netherlands 11 Department of Physics, Bergische Universität Wuppertal, Wuppertal, Germany 12 Institut für Prozessdatenverarbeitung und Elektronik, Karlsruhe Institute of Technology (KIT), Karlsruhe, Germany 13 Faculty of Natural Sciences and Engineering, Universität Siegen, Siegen, Germany 14 Institute of Space Science, Bucharest-Magurele, Romania 15 Department of Astrophysics, National Centre for Nuclear Research, Łód´z, Poland 16 Studsvik Scandpower GmbH, Hamburg, Germany 17 Department of Physics and Astronomy, Bartol Research Institute, University of Delaware, Newark, DE, USA 18 Department of Physics, University of Bucharest, Bucharest, Romania 19 Present address European Commission, Joint Research Centre (JRC), Karlsruhe, Germany Received: 10 June 2020 / Accepted: 25 January 2021 / Published online: 22 February 2021 © The Author(s) 2021 Abstract LOPES, the LOFAR prototype station, was an Technology (KIT). As a prototype experiment, LOPES tested antenna array for cosmic-ray air showers operating from several antenna types, array configurations and calibration 2003 to 2013 within the KASCADE-Grande experiment. techniques, and pioneered analysis methods for the recon- Meanwhile, the analysis is finished and the data of air-shower struction of the most important shower parameters, i.e., the events measured by LOPES are available with open access in arrival direction, the energy, and mass-dependent observables the KASCADE Cosmic Ray Data Center (KCDC). This arti- such as the position of the shower maximum. In addition to cle intends to provide a summary of the achievements, results, a review and update of previously published results, we also and lessons learned from LOPES. By digital, interferometric present new results based on end-to-end simulations includ- beamforming the detection of air showers became possible ing all known instrumental properties. For this, we applied in the radio-loud environment of the Karlsruhe Institute of the detector response to radio signals simulated with the CoREAS extension of CORSIKA, and analyzed them in the same way as measured data. Thus, we were able to study the a e-mail: [email protected] detector performance more accurately than before, including b e-mail: [email protected] (corresponding author) 123 176 Page 2 of 25 Eur. Phys. J. C (2021) 81 :176 some previously inaccessible features such as the impact of With improved methods and at more radio-quiet sites, the noise on the interferometric cross-correlation beam. These successor arrays LOFAR in the Netherlands [18], the Auger results led to several improvements, which are documented Engineering Radio Array (AERA) of the Pierre Auger Obser- in this paper and can provide useful input for the design of vatory in Argentina [19], and Tunka-Rex in Siberia [20]have future cosmic-ray experiments based on the digital radio- meanwhile achieved an accuracy comparable to the leading detection technique. optical techniques for air showers even for the position of the shower maximum. In general, radio detection combines several advantages 1 Introduction of the classical detection techniques for air showers. Like the air-fluorescence and air-Cherenkov light of air showers, also What is the potential of the digital radio detection technique the radio emission depends on the relatively well-understood for high-energy astroparticle physics? To answer this ques- electromagnetic shower component, i.e., the radio signal has tion, LOPES (the LOFAR PrototypE Station) was deployed an intrinsic sensitivity to the energy of the electromagnetic in the early 2000s and operated for about one decade. Mean- component and the position of the shower maximum. The while the experiment is concluded and data analysis finished. radio technique is not restricted to clear nights, but works Therefore, it is time to summarize: What is the answer to the under almost any weather conditions. LOPES showed that original question? What has been achieved? What else have only thunderclouds directly over the antenna array have we learned, what new questions emerged, and how is the field a significant impact on the radio signal [21], which low- continuing? ers the available measurement time by only a few percent. Thanks to prototype experiments such as LOPES, digital Radio detectors share their advantage of availability around radio detection has been established as an additional tech- the clock with the classical technique of secondary-particle nique for the measurement of cosmic-ray air showers. First detection at the ground. Thus, antennas arrays are the ideal measurements of the radio emission were performed with companion to particle detectors, in particular to muon detec- analog detectors more than fifty years ago [1–3]. However, tors that yield complementary information to the electro- their accuracy for the cosmic-ray energy and the position of magnetic shower component measured via the radio signal. the shower maximum was limited by the analog technology Moreover, as it was done at LOPES, a particle detector array used and by the incomplete theoretical understanding of the can provide a trigger to the radio antennas, which facilitates radio emission of air showers. Nevertheless, the community the discrimination of air-shower radio signal against back- maintained a certain level of interest in the technique and ground. continued experimental efforts regarding the radio detection Digital radio experiments [22–24] also revealed some of air showers [4,5]. A technical breakthrough was achieved complications of the radio technique: due to the interplay in the 2000’s with the digital antenna arrays LOPES [6] and of two different emission mechanisms, the radio footprint on CODALEMA [7] because the digitally saved data enabled ground has a two-dimensional, asymmetric shape. The two sophisticated post-processing and computing-extensive anal- proven emission mechanisms are the geomagnetic deflection yses of the measurements. In parallel, progress was made on of the electrons and positrons [25,26], which is the domi- a variety of simulation and calculation tools [8–11], whose nant effect for most air-shower geometries, and the weaker latest generations are generally able to reproduce measured Askaryan effect, i.e., radio emission due to the variation of radio signals. Together with the second generation of digital the net charge of the shower front during the shower develop- antenna arrays such as AERA [12], LOFAR [13], TREND ment [27]. Several radio arrays in radio-quiet environments [14], and Tunka-Rex [15], the measurements of LOPES and have experimentally confirmed the coexistence of these two CODALEMA were critical to improve the understanding of emission mechanisms [28–30]. At LOPES, this complica- the emission processes, in particular by comparing the mea- tion was neglected against the typical measurement uncer- sured radio signals with the predictions of Monte Carlo sim- tainties, i.e., the LOPES measurements were interpreted as ulations of extensive air showers and to the data of the co- if the radio emission were purely geomagnetic. The result- located particle detector arrays. ing inaccuracy was small compared to other uncertainties, Because LOPES was triggered by the co-located particle- e.g., resulting from the high radio background at the site. detector array KASCADE-Grande, a comparison of radio Another complication of the radio technique regards self- and particle measurements of the same air showers demon- triggering, which turned out to be possible, but difficult, strated that radio detection provides an accuracy for the and is unnecessary when radio arrays are operated as exten- shower direction [16] and energy [17] approximately equal to sion to particle-detector arrays. Finally,
Details
-
File Typepdf
-
Upload Time-
-
Content LanguagesEnglish
-
Upload UserAnonymous/Not logged-in
-
File Pages25 Page
-
File Size-