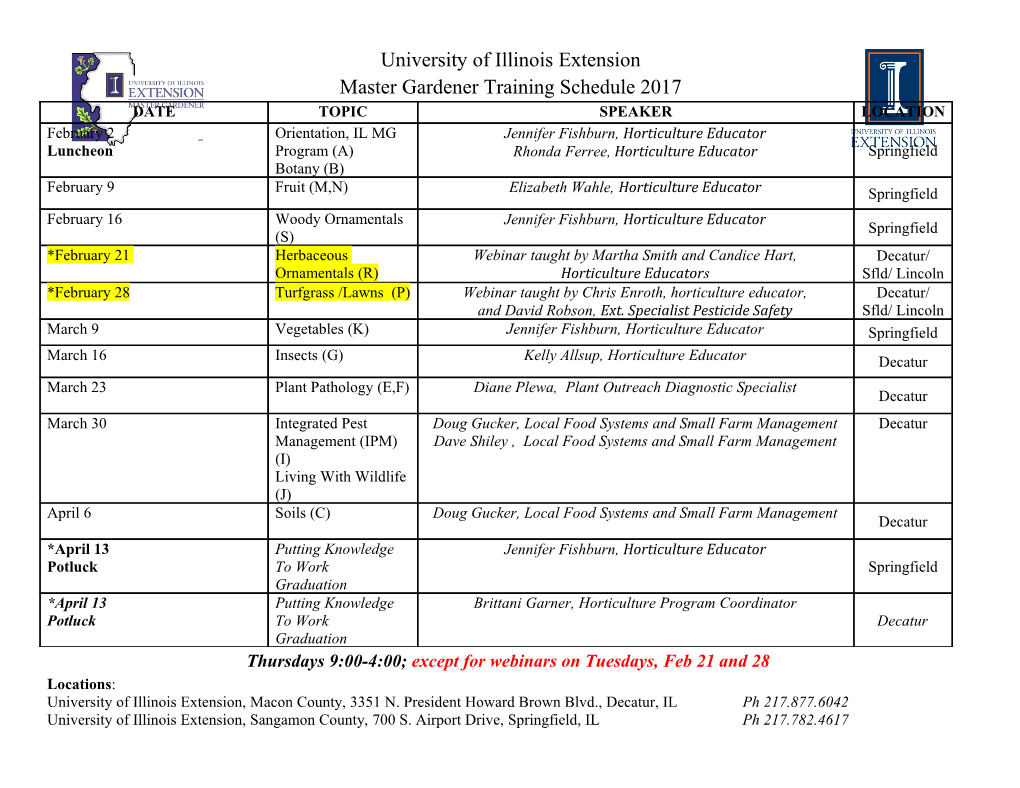
858 IEEE JOURNAL OF SELECTED TOPICS IN QUANTUM ELECTRONICS, VOL. 11, NO. 4, JULY/AUGUST 2005 Optical Trapping and Coherent Anti-Stokes Raman Scattering (CARS) Spectroscopy of Submicron-Size Particles James W. Chan, Heiko Winhold, Stephen M. Lane, and Thomas Huser Abstract—Optical trapping combined with coherent anti-Stokes (∼ 10−30 cm2) that limit its practical use for imaging applica- Raman scattering (CARS) spectroscopy is demonstrated for the tions. The orders of magnitude higher signals in CARS mi- first time as a new technique for the chemical analysis of individ- croscopy allow for rapid CARS imaging approaching video rate ual particles over an extended period of time with high temporal resolution. Single submicron-size particles suspended in aqueous speed over long time periods, which enables monitoring of rapid media are optically trapped and immobilized using two tightly dynamic processes [5], [6] that are otherwise not feasible with focused collinear laser beams from two pulsed Ti:Sapphire laser conventional fluorescence or Raman microscopy. sources. The particles can remain stably trapped at the focus for In the CARS technique, two laser beams at two different many tens of minutes. The same lasers generate a CARS vibra- frequencies (ω , the pump frequency and ω , the Stokes fre- tional signal from the molecular bonds in the trapped particle when p s the laser frequencies are tuned to a vibrational mode of interest, quency) are tightly focused into the sample to generate a co- providing chemical information about the sample. The technique herent anti-Stokes signal at frequency 2ωp − ωs . Strong reso- is characterized using single polystyrene beads and unilamellar nant CARS signals are generated when the frequency differ- phospholipid vesicles as test samples and can be extended to the ence ωp − ωs matches a Raman-active molecular vibration in study of living biological samples. This novel method could poten- the sample, which induces collective oscillations of the molec- tially be used to monitor rapid dynamics of biological processes in single particles on short time scales that cannot be achieved by ular bonds. Because CARS is a nonlinear multiphoton pro- using other vibrational spectroscopy techniques. cess, the signal is only generated within the focal volume, providing high spatial resolution akin to other multiphoton Index Terms—Biophotonics, coherent anti-Stokes Raman scat- tering (CARS), laser tweezers, Raman spectroscopy. techniques, such as two-photon fluorescence and second har- monic generation. A CARS image is generated by rapid scan- ning of the laser focus over the entire biological sample. An I. INTRODUCTION additional benefit of CARS microscopy is that sample aut- OHERENT anti-Stokes Raman scattering (CARS) mi- ofluorescence does not usually interfere with the CARS sig- C croscopy has been developed recently [1]–[4] as a tech- nal, which is located at wavelengths shorter than the excitation nique capable of high spatial resolution for the chemical imag- wavelengths. ing of live biological samples without the need for intrusive The long-term CARS analysis of submicron-size biological labeling with fluorescent probes. Contrast in CARS imaging particles suspended in aqueous media is difficult, if not impos- is generated from vibrational signals of Raman active molec- sible, due to the constant Brownian motion of the particle in ular vibrations in the sample itself. Consequently, a major and out of the laser focus. Optical tweezers [7]–[11] have been benefit of CARS imaging is that the signal, unlike the sig- shown to be able to immobilize a particle in solution such that nal from fluorescent probes, does not photobleach, enabling it remains within the focal volume of the focused laser beam, long-term imaging of a living sample as it undergoes biolog- essentially “trapping” the particle indefinitely. This trapping is ical processes. The coherent nature of the nonlinear CARS enabled by photons from the laser beam imparting their mo- process results in vibrational signals that are much stronger mentum to the particle, which results in transverse and axial than spontaneous Raman signals. Spontaneous Raman spec- forces that are balanced near the laser focus. Several more re- troscopy is known for its relatively low scattering cross-sections cent studies [12]–[18] have already demonstrated the ability to combine optical trapping with spontaneous Raman spectroscopy using continuous wave (CW) laser sources, which allows for the Manuscript received December 17, 2004; revised June 15, 2005. This work chemical analysis of biological specimens and their dynamics. was supported by the National Science Foundation (NSF). The Center for Bio- However, this laser tweezers Raman spectroscopy (LTRS) tech- photonics, an NSF Science and Technology Center, is managed by the University of California, Davis, under Cooperative Agreement No. PHY 0120999. Work at nique still suffers from the inability to acquire spectra rapidly LLNL was performed under the auspices of the U.S. Department of Energy by enough to monitor fast dynamics. Typically, spectra have to the University of California, Lawrence Livermore National Laboratory, under be acquired over periods of many seconds to minutes, thus al- Contract No. W-7405-Eng-48. The authors are with Lawrence Livermore National Laboratory, Livermore, lowing only slow, long-term dynamics (e.g., apoptosis) to be CA 94550 USA, and also with the NSF Center for Biophotonics Science studied. CARS signals, in contrast, being that much stronger and Technology, University of California-Davis, Sacramento, CA 95817 USA than spontaneous Raman signals, would enable monitoring of (e-mail: [email protected]; [email protected]; [email protected]; huser1@ llnl.gov). fast dynamics with higher temporal resolution and maintaining Digital Object Identifier 10.1109/JSTQE.2005.857381 of chemical specificity. 1077-260X/$20.00 © 2005 IEEE CHAN et al.: OPTICAL TRAPPING AND (CARS) SPECTROSCOPY OF SUBMICRON-SIZE PARTICLES 859 spectrometer (Ocean Optics, Dunedin, FL) with a resolution of roughly 1 nm. The parallel-polarized beams are expanded with a telescope assembly to approximately 5 mm and sent into an inverted optical microscope (Axiovert 200, Zeiss) equipped with a dichroic mirror and a 100 ×, 1.3 NA oil immersion objective (Plan-NEOFLUAR, Zeiss, Germany). CARS signals generated at the laser focus are epi-detected and sent through a 100-µm confocal pinhole and a short pass filter for rejection of the excitation light. The CARS signal is detected with either an avalanche photodiode or a grating spectrometer equipped with a liquid nitrogen cooled 1340 × 100 pixel charge-coupled device (CCD) camera (Roper Scientific, Trenton, NJ). The system is also equipped with a separate 30-mW He-Ne laser at 633 nm that is capable of trapping single particles and functions as an excitation laser for acquiring spontaneous Raman spectra. In addition, a pulse picker (NEOS Technologies, Melbourne, Fig. 1. Schematic of the Raman and CARS confocal detection system. Two FL) can be added right after both beams are combined by the pulsed Ti:Sapphire laser beams are collinearly combined into an inverted mi- croscope. Detection of the spectral signals is achieved using a standard confocal dichroic reflector to reduce the repetition rate of each laser geometry, an avalanche photodiode, and spectrometer. A CW He-Ne laser is beam down to 200 kHz. This reduces the average power of also implemented for spontaneous Raman measurements, and a pulse picker is the beams and minimizes photodamage to samples, while used to reduce the repetition rate of the Ti:Sapphire beams. maintaining high peak powers for CARS signal generation. Optical trapping with pulsed laser systems has not been as B. Preparation of Unilamellar Vesicles widely demonstrated as with CW lasers but has been gaining 1,2-Dimyristoyl-sn-glycero-3-phosphocholine (DMPC) is considerable attention due to the ability to study a trapped purchased in powder form from Avanti Polar Lipids, Alabaster, particle by nonlinear spectroscopic methods that require intense AL. Liposomes are prepared using standard techniques [23]. short laser pulses. Modeling studies [19] have predicted the Briefly, a DMPC/chloroform solution is dried under nitrogen for feasibility of ultrashort-pulsed laser tweezers, and experimental several minutes, placed under vacuum overnight to remove trace studies have demonstrated the combination of trapping with quantities of chloroform, and hydrated above its lipid transition SHG [20], [21] and two-photon fluorescence [22]. In this temperature. Unilamellar vesicles are prepared through extru- paper, we demonstrate the combination of optical trapping with sion of the multilamellar vesicle solution using a mini-extruder CARS spectroscopy using two pulsed laser sources at different (Avanti Polar Lipids). Approximately 1 mL of the hydrated lipid wavelengths that are overlapped both spatially and temporally. suspension is extruded several times at its phase transition tem- This technique makes it possible to rapidly obtain chemical perature using polycarbonate membranes (Avanti Polar Lipids) information from submicron-size particles in their natural with a 0.4-µm pore size to yield a concentrated solution of environment. The trapping parameters and CARS signal are 0.4-µm diameter unilamellar vesicles. This solution is then di- demonstrated and fully characterized for polystyrene bead and luted several times to a point where single vesicles can be ob- unilamellar vesicle samples. served and trapped
Details
-
File Typepdf
-
Upload Time-
-
Content LanguagesEnglish
-
Upload UserAnonymous/Not logged-in
-
File Pages6 Page
-
File Size-