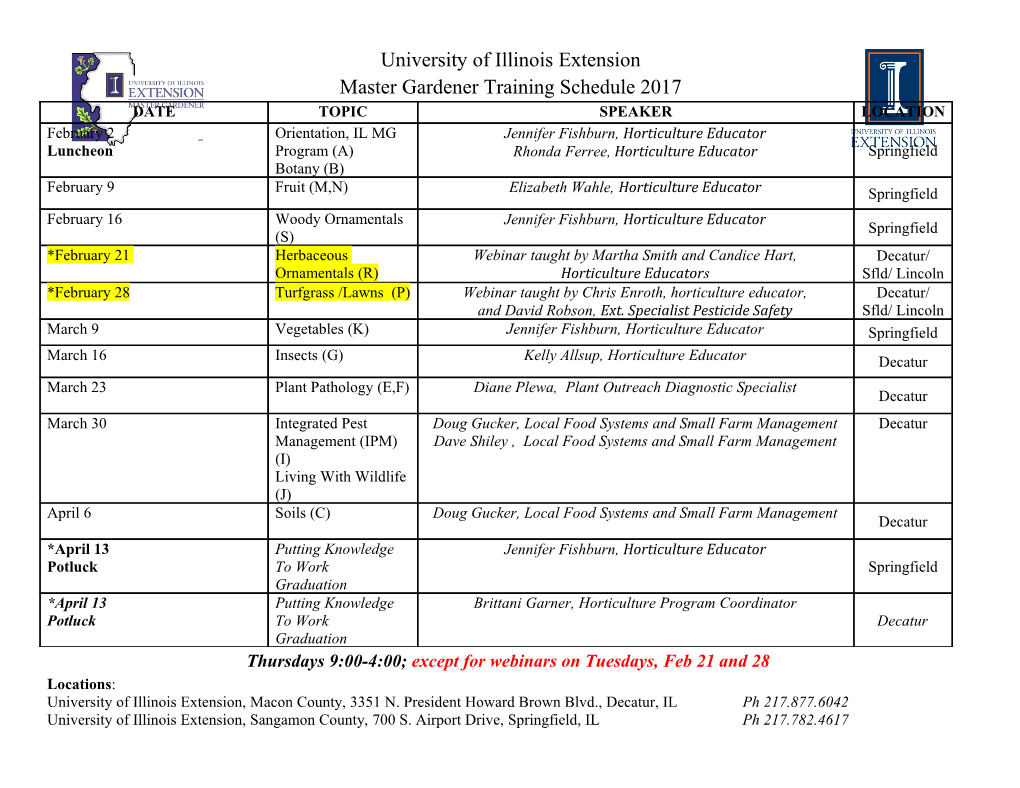
applied sciences Review Recent Advancements and Future Prospects of Noble Metal-Based Heterogeneous Nanocatalysts for Oxygen Reduction and Hydrogen Evolution Reactions Dinesh Bhalothia 1, Lucky Krishnia 2, Shou-Shiun Yang 1, Che Yan 1 , Wei-Hao Hsiung 1, Kuan-Wen Wang 3 and Tsan-Yao Chen 1,4,5,6,* 1 Department of Engineering and System Science, National Tsing Hua University, Hsinchu 30013, Taiwan; [email protected] (D.B.); [email protected] (S.-S.Y.); [email protected] (C.Y.); [email protected] (W.-H.H.) 2 Amity Centre of Nanotechnology, Amity University Haryana, Panchgaon, Gurugram-122413, India; [email protected] 3 Institute of Materials Science and Engineering, National Central University, Taoyuan City 32001, Taiwan; [email protected] 4 Institute of Nuclear Engineering and Science, National Tsing Hua University, Hsinchu 30013, Taiwan 5 Hierarchical Green-Energy Materials (Hi-GEM) Research Centre, National Cheng Kung University, Tainan 70101, Taiwan 6 Department of Materials Science and Engineering, National Taiwan University of Science and Technology, Taipei 10617, Taiwan * Correspondence: [email protected]; Tel.: +886-3-5715131 or +885-3-5720724 Received: 28 September 2020; Accepted: 28 October 2020; Published: 30 October 2020 Abstract: The oxygen reduction reaction (ORR) and hydrogen evolution reaction (HER) both are key electrochemical reactions for enabling next generation alternative-power supply technologies. Despite great merits, both of these reactions require robust electrocatalysts for lowering the overpotential and promoting their practical applications in energy conversion and storage devices. Although, noble metal-based catalysts (especially Pt-based catalysts) are at the forefront in boosting the ORR and HER kinetics, high cost, limited availability, and poor stability in harsh redox conditions make them unfit for scalable use. To this end, various strategies including downsizing the catalyst size, reducing the noble metal, and increasing metal utilization have been adopted to appropriately balance the performance and economic issues. This mini-review presents an overview of the current state of the technological advancements in noble metal-based heterogeneous nanocatalysts (NCs) for both ORR and HER applications. More specifically, we focused on establishing the structure–performance correlation. Keywords: hydrogen evolution reaction; nanocatalysts; electrochemical catalysis 1. Introduction The continuously increasing imbalance between energy supply and demand along with adverse climatic issues have reinforced the importance of implementing a sustainable and environmentally benign energy economy. Recently, the International Energy Agency reported that the global energy demand will increase by nearly 30% by the end of 2040. Meanwhile, the CO2 emissions will hit 1 35.7 Gt year− by 2040 [1]. In this context, fuel cells are believed to be an ultimate solution for the future energy supply owing to their high energy conversion efficiency with zero emissions [2]. However, the sluggish kinetics of the oxygen reduction reaction (ORR) on the cathode side is a major hurdle for their practical and scalable usage; hence, we need highly efficient electrocatalysts to boost ORR kinetics [3]. At the same time, hydrogen (H2) has also emerged as an ultimate ideal energy carrier Appl. Sci. 2020, 10, 7708; doi:10.3390/app10217708 www.mdpi.com/journal/applsci Appl. Sci. 2020, 10, 7708 2 of 19 1 by virtue of it having the highest gravimetric energy density (142 MJ kg− ) of all the carbonaceous species and zero emissions [4]. On Earth, hydrogen is not found in free form and is mainly available in compounds (e.g., hydrocarbons and water), hence, the effective production of hydrogen is of paramount importance for the implementation of the hydrogen economy. At present, nearly 95% of the total hydrogen is produced by coal gasification and steam reforming, while only 4% is obtained from water splitting [5]. Currently, hydrogen production through non-renewable resources (i.e., fossil fuels) severely threatens the environment via massive CO2 emission [6]. In addition, steam-methane reforming is an intensive energy consumption process, in which hydrocarbon and water react together at high-temperature to generate CO2 and hydrogen via the following reactions: CH + H O CO + 3H (1) 4 2 ! 2 CO + H O CO + H (2) 2 ! 2 2 In these reactions, every mole of hydrogen produces one mole of CO2. Neither of these methods for hydrogen production support the reduction of environmental pollution and global warming and, therefore, they are not environmentally benign processes for sustainable hydrogen production. Although electrochemical water splitting is an effective approach to generate cheap and sustainable hydrogen at a large scale, the relatively suppressed efficiency and the lack of efficient, economical, and earth-abundant electrocatalysts for the hydrogen evolution reaction (HER) are major hurdles in this step [7,8]. Electrochemical water splitting was first observed in 1789 yet contributes only 4% of the H2 production worldwide, even after 200 years of development, due to sluggish kinetics of HER at the cathode in water splitting [9]. Nevertheless, similar to ORR, the HER also has to surmount a certain energy barrier (known as overpotential) to occur. Generally, for any electrochemical reaction, the difference between the applied and thermodynamic potentials is termed as overpotential. In this event, for lowering the overpotential and promoting the reaction rate, both ORR and HER usually require the assistance of electrocatalysts. To date, noble metals, particularly Pt-based electrocatalysts, are highly utilized in the practice of boosting the ORR and HER kinetics [10]. However, Pt is restricted by its high cost and scarcity for industry applications [11]. Therefore, the development of highly efficient electrocatalysts with reduced Pt-loading and high utilization is an urgent need for the establishment of a carbon-neutral economy. Tremendous efforts have been directed in the past decades to potentially address the aforementioned issues. Recent pioneer studies have demonstrated the effective approach to reduce noble metal usage via scaling down the catalyst size to clusters or single atoms [12], incorporating the noble metals with cheap transition metals in the form of alloys [13], and epitaxial or core-shell nanostructures [14,15]. In addition, several studies have highlighted noble metal-free catalysts for ORR and HER including transition metal chalcogenides [16], nitrides [17], phosphides [18], carbides [19], and borides [20]. Moreover, the development of effective metal-free ORR and HER catalysts are also underway [21]. Despite great endeavors, noble metal-free catalysts are still in their infant stage and are not competitive to Pt-based catalysts. Keeping all this in view, herein, we present a mini-review of ORR and HER electrocatalysts, mainly emphasizing noble metal-based heterogeneous nanocatalysts (NCs). 2. Reaction Pathways For designing omnipotent ORR and HER catalysts, it is important to understand how these electrochemical reactions proceed on a catalyst surface. The mechanistic details of ORR and HER are given in the following section. 2.1. Mechanism of ORR The ORR is a complex multi-electron reaction that may include several elementary steps involving different reaction intermediates. The mechanism of ORR is not fully elucidated due to the complexity and changing behavior of the process depending upon the electrode materials, catalysts, and the Appl. Sci. 2020, 10, 7708 3 of 19 electrolyte. However, the literature says that the ORR can proceed either via a direct four-electron transfer route or via a series of two-electron transfer routes irrespective of the electrolyte; the two-electron pathway leads to hydrogen peroxide (H2O2) production and the four-electron transfer route results in water (H2O) production. The four-electron transfer process is generally preferred because H2O2 damages the Nafion membrane in fuel cells. The following equations illustrate both of the pathways when using acidic or alkaline electrolytes: Acidic electrolyte: + O + 4H + 4e− 2H O (3) 2 ! 2 + O + 2H + 2e− H O (4) 2 ! 2 2 + H O + 2H + 2e− 2H O (5) 2 2 ! 2 Alkaline electrolyte: O + H O + 4e− 4OH− (6) 2 2 ! O + H O + 2e+ HO− + OH− (7) 2 2 ! 2 HO− + H O + 2e− 3OH− (8) 2 2 ! 2.2. Mechanism of HER The understanding of the HER mechanism is a key factor for designing the next generation HER catalysts. Depending on the pH values of the electrolyte solution, HER proceeds through the + reduction of protons (H ) or water (H2O) molecules accompanied by the subsequent evolution of gaseous hydrogen (Figure1). Figure 1. The hydrogen evolution reaction (HER) pathways on the catalyst surface. Appl. Sci. 2020, 10, 7708 4 of 19 In acidic media (i.e., pH < 7.0), the HER involves two successive steps on the surface of the catalyst. The discharge or Volmer reaction takes place in the first step of HER. In this step, a proton (H+) is adsorbed on the empty active site of the NC surface to yield an adsorbed hydrogen atom ads + (H ) (Equation (9)). The hydronium cation (i.e., H3O ) is the proton source in the acidic medium. In the subsequent step, a second proton (i.e., H+) combines with an adsorbed hydrogen atom (Hads; from step 1) and an electron (e−) to evolve H2 (Equation (10)). This step is known as the Heyrovsky step or electrochemical desorption step or ion + atom step. Herein, H2 formation may also occur via an alternative pathway, which is known as Tafel or chemical desorption step. In this pathway, H2 is produced via a combination of two Hads on the NC surface (Equation (11)). + ads H O + e− H + H O (9) 3 ! 2 ads + H + H O + e− H + H O (10) 3 ! 2 2 2Hads H (11) ! 2 The HER is relatively slower in an alkaline medium (i.e., pH > 7.0) due to the lack of protons. In an alkaline medium, the HER starts by dissociating water (i.e., H2O) molecules to generate protons. The dissociation of H2O is involved in both the Volmer (Equation (12)) and Heyrovsky (Equation (13)) steps during HER in an alkaline medium.
Details
-
File Typepdf
-
Upload Time-
-
Content LanguagesEnglish
-
Upload UserAnonymous/Not logged-in
-
File Pages19 Page
-
File Size-