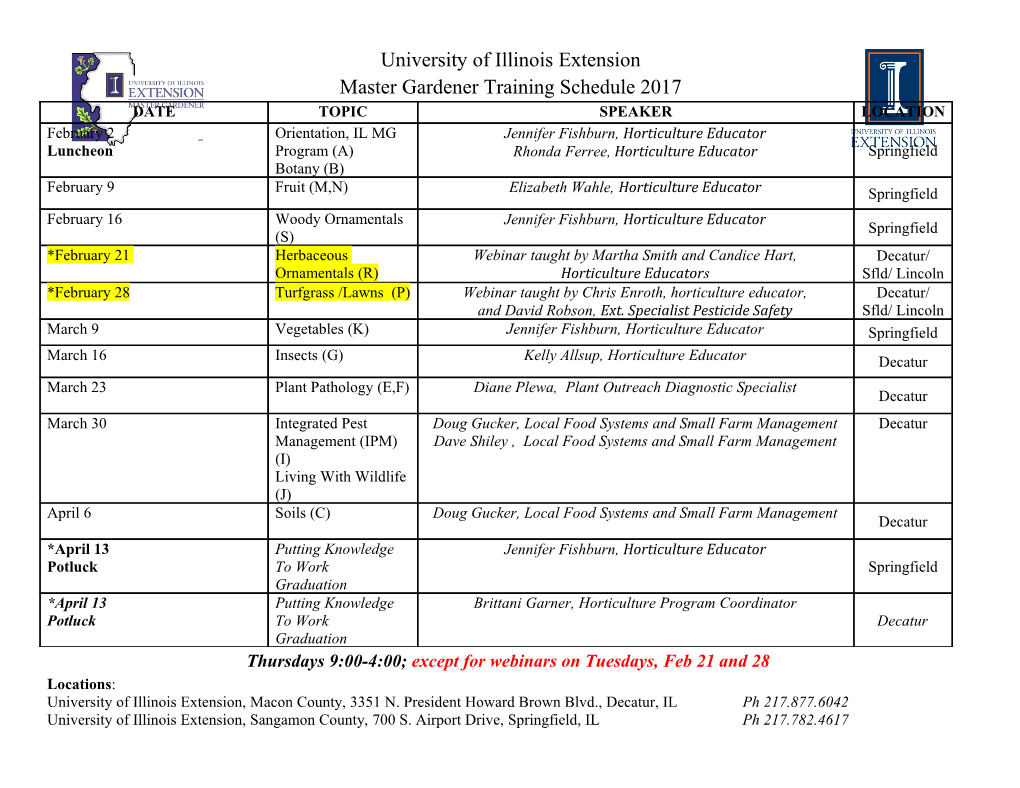
Pacing of Paleozoic macroevolutionary rates by Milankovitch grand cycles James S. Cramptona,b,1, Stephen R. Meyersc, Roger A. Coopera, Peter M. Sadlerd, Michael Footee, and David Hartef aDepartment of Paleontology, GNS Science, 5040 Lower Hutt, New Zealand; bSchool of Geography, Environment and Earth Sciences, 6140 Wellington, New Zealand; cDepartment of Geoscience, University of Wisconsin–Madison, Madison, WI 53706; dDepartment of Earth Sciences, University of California, Riverside, CA 92521; eDepartment of the Geophysical Sciences, University of Chicago, Chicago, IL 60637; and fDepartment of Tectonophysics, GNS Science, 5040 Lower Hutt, New Zealand Edited by Charles R. Marshall, University of California, Berkeley, CA, and accepted by Editorial Board Member Douglas Futuyma March 30, 2018 (received for review August 15, 2017) Periodic fluctuations in past biodiversity, speciation, and extinc- g4-g3, the orbital perihelion precession rates of Mars and Earth, tion have been proposed, with extremely long periods ranging and s4-s3, the orbital inclination rates of Mars and Earth, re- from 26 to 62 million years, although forcing mechanisms remain spectively. These grand cycles have been implicated as controls speculative. In contrast, well-understood periodic Milankovitch on Late Cenozoic ice sheet history (10) and sea-level variability climate forcing represents a viable driver for macroevolutionary into the Mesozoic (11). The environmental impact of the grand fluctuations, although little evidence for such fluctuation exists cycles is to produce long-term “nodes” of stability (e.g., little dif- except during the Late Cenozoic. The reality, magnitude, and ference in climate between maximum and minimum of obliquity) drivers of periodic fluctuations in macroevolutionary rates are of that alternate with times of maximum volatility (e.g., strong cli- interest given long-standing debate surrounding the relative roles matic differences between maximum and minimum of obliquity). of intrinsic biotic interactions vs. extrinsic environmental factors as Whereas this multimillion year control on environmental stability drivers of biodiversity change. Here, we show that, over a time has obvious implications for biological evolution, its presence has span of 60 million years, between 9 and 16% of the variance in not been clearly detected in evolutionary rate data, except in the biological turnover (i.e., speciation probability plus species extinc- case of the Neogene mammalian record (6). A major obstacle in tion probability) in a major Early Paleozoic zooplankton group, the this regard has been the availability of records of appropriate graptoloids, can be explained by long-period astronomical cycles duration and sampling frequency to permit a robust evaluation. (Milankovitch “grand cycles”) associated with Earth’s orbital ec- Graptoloids (order Graptoloidea) are an extinct group of co- centricity (2.6 million years) and obliquity (1.3 million years). These lonial, filter-feeding hemichordates that constituted the main grand cycles modulate climate variability, alternating times of rel- component of the Paleozoic macrozooplankton as preserved in ative stability in the environment with times of maximum volatil- the fossil record (12, 13) from the beginning of the Ordovician ity. We infer that these cycles influenced graptolite speciation and Period (486 Ma) to the Early Devonian (411 Ma) (14, 15). They have a very short median species life span—1.27 and 0.69 My in extinction through climate-driven changes to oceanic circulation — and structure. Our results confirm the existence of Milankovitch the Ordovician and Silurian, respectively (16) meaning that grand cycles in the Early Paleozoic Era and show that known pro- they provide a rich dataset for analysis of speciation and ex- cesses related to the mechanics of the Solar System were shaping tinction rates (Fig. 2). For this reason and due to their abun- marine macroevolutionary rates comparatively early in the history dance and the resultant highly resolved record, they provide a of complex life. We present an application of hidden Markov models model clade to investigate million year-scale astronomical influ- ence on macroevolution during the Paleozoic. to macroevolutionary time series and protocols for the evaluation of statistical significance in spectral analysis. Significance Paleozoic | graptoloids | Milankovitch grand cycles | macroevolution | macroevolutionary rates There has been long-standing debate about the relative roles of intrinsic biotic interactions vs. extrinsic environmental factors as he relative roles of intrinsic biotic interactions vs. extrinsic drivers of biodiversity change. Here, we show that, relatively “ ” Tenvironmental factors as drivers of biodiversity change have early in the history of complex life, Milankovitch grand cycles been much debated and are still uncertain (1, 2). One facet of this associated with astronomical rhythms explain between 9 and debate concerns the reality and causes of putative periodic fluctu- 16% of variation in species turnover probability (extinction ations in diversity, speciation rate, and extinction rate. In particular, probability plus speciation probability) in a major Early Paleozoic zooplankton group, the graptoloids. These grand cycles would quasiregular fluctuations with extremely long periods ranging from have modulated climate variability, alternating times of relative 26 (3) to 62 (4) My have been proposed, although the forcing stability in the environment with times of maximum volatility, mechanisms have remained speculative (5). With the exception of which influenced oceanic circulation and structure and thus, the Late Cenozoic (6), no studies have shown the role of well- phytoplankton populations at the base of the marine food web. understood astronomical cycles on rates of evolution or extinction or quantified the proportion of variance in macroevolutionary Author contributions: J.S.C., S.R.M., and R.A.C. designed research; R.A.C. and P.M.S. gener- time series that can be explained by these cycles. Here, we show ated the CONOP composite; J.S.C. and D.H. generated the hidden Markov models; S.R.M. that long-period astronomical cycles, Milankovitch “grand cycles,” undertook spectral analyses; J.S.C., S.R.M., R.A.C., P.M.S., M.F., and D.H. interpreted results; played a significant role in pacing species turnover in a major Early and J.S.C., S.R.M., R.A.C., P.M.S., M.F., and D.H. wrote the paper. Paleozoic zooplankton group, the graptoloids. The authors declare no conflict of interest. Milankovitch grand cycles (7) are astronomical rhythms as- This article is a PNAS Direct Submission. C.R.M. is a guest editor invited by the sociated with the amplitude modulation of Earth’s climatic Editorial Board. precession cycle and axial obliquity cycle. During the Late Published under the PNAS license. Cenozoic, the amplitude modulation of precession by eccen- 1To whom correspondence should be addressed. Email: [email protected]. tricity results in a 2.4-My cycle in addition to the well-known This article contains supporting information online at www.pnas.org/lookup/suppl/doi:10. cycles of 405,000 and ∼100,000 y; the long-period obliquity 1073/pnas.1714342115/-/DCSupplemental. amplitude modulation is ∼1.2 My (Fig. 1) (8, 9). These relate to Published online May 14, 2018. 5686–5691 | PNAS | May 29, 2018 | vol. 115 | no. 22 www.pnas.org/cgi/doi/10.1073/pnas.1714342115 Downloaded by guest on September 30, 2021 A node node node node 0.00 0.04 Climatic precession -0.04 B Radians 0.39 0.41 10 8 6 4 2 Ma Fig. 1. Illustrations of Milankovitch grand cycles over the past 1–11 My generated from the astronomical solution in ref. 9. (A) Amplitude modulation of precession by eccentricity. The black line is the climatic precession [= eccentricity × sin(precession angle)]; the red line is the amplitude modulation and reveals cycles at ∼100,000 and ∼405,000 y and grand cycle nodes at ∼2.4 My. (B) Amplitude modulation of obliquity. The black line is the obliquity solution; the red line is the amplitude modulation of the dominant 41,000-y obliquity cycle, with a conspicuous ∼1.2-My grand cycle. Graptoloid colony size generally ranged from a few millime- Results ters up to ∼200 mm in maximum dimension, although the indi- The time series of graptolite HMM species turnover (speciation vidual zooids measured less than 2 mm in length (20). The plus extinction HMM probabilities) reveals a strong 2.6-My colonies lived suspended in the ocean waters at a range of depth rhythm expressed in both the time frequency result (Figs. 2 C zones and are inferred to have filtered out microphytoplankton, and D and 3 and Figs. S3 and S5) and power spectrum for the bacterioplankton, and other particulate organic matter as their entire study interval (Fig. S2); this rhythm is close to that of the ∼ principal food source (21–23). The graptoloids are, therefore, modern day orbital eccentricity grand cycle. In contrast, a 1.3-My inferred to have been primary consumers in the food chain and rhythm that is weak in the total spectrum is dominant in the early portion of the record, with a transition between the two in the consequently, would have been sensitive to environmental param- interval from 460 to 453 Ma (Figs. 2 C and D and 3 and Fig. S4). eters that controlled their main trophic resource, namely nutrient This signal is close to that of the modern day obliquity grand cycle. flux, ocean stratification and chemistry, redox profile, local and Together, these two cycles explain between 9 and 16% of the total global ocean circulation systems, and therefore, global marine cli- variance in the turnover signal (Fig. 3A). Both rhythms are sta- mate (16, 21, 24, 25). In support of this environmental sensitivity, tistically significant. The testing of statistical significance in time 13 positive excursions in the global δ Ccarb isotope curve—interpreted series analysis is a complex issue that is addressed in detail in as reflecting carbon burial and associated global cooling (26–28)— Materials and Methods and Supporting Information.
Details
-
File Typepdf
-
Upload Time-
-
Content LanguagesEnglish
-
Upload UserAnonymous/Not logged-in
-
File Pages6 Page
-
File Size-