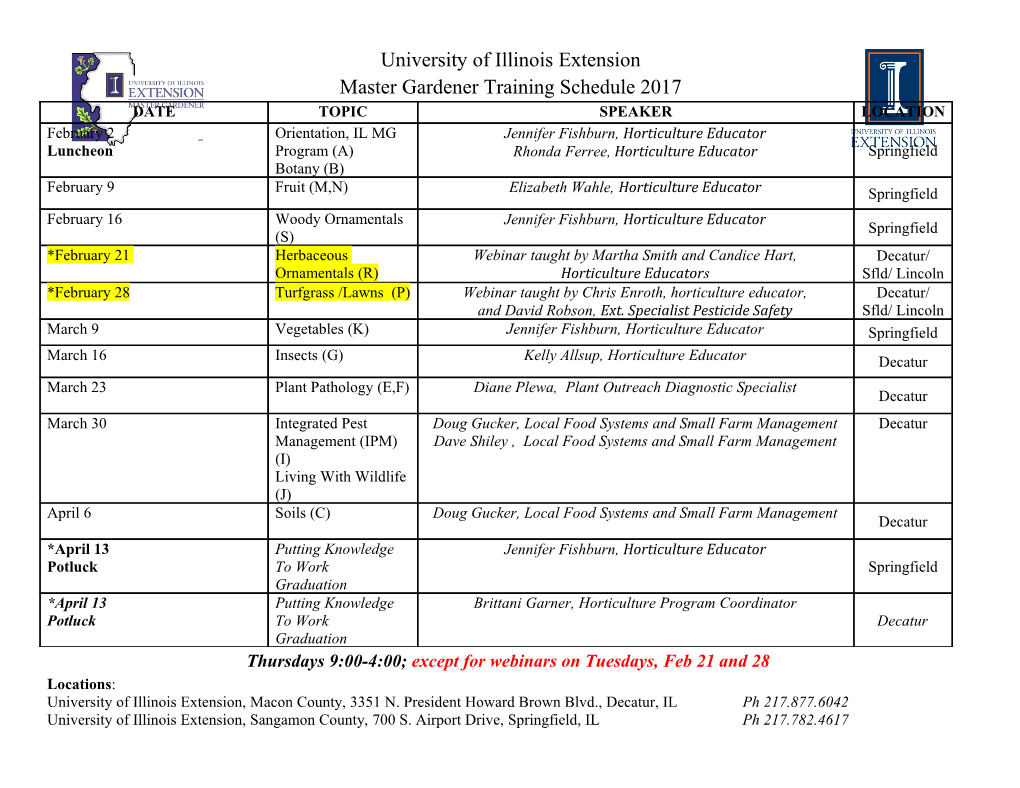
batteries Review Review of Multivalent Metal Ion Transport in Inorganic and Solid Polymer Electrolytes Lauren F. O’Donnell and Steven G. Greenbaum * Department of Physics and Astronomy, Hunter College of the City University of New York, New York, NY 10065, USA; [email protected] * Correspondence: [email protected]; Tel.:+1-212-772-4973 Abstract: The lithium ion battery, with its high energy density and low reduction potential, continues to enchant researchers and dominate the landscape of energy storage systems development. However, the demands of technology in modern society have begun to reveal limitations of the lithium energy revolution. A combination of safety concerns, strained natural resources and geopolitics have inspired the search for alternative energy storage and delivery platforms. Traditional liquid electrolytes prove precarious in large scale schemes due to the propensity for leakage, the potential for side reactions and their corrosive nature. Alternative electrolytic materials in the form of solid inorganic ion conductors and solid polymer matrices offer new possibilities for all solid state batteries. In addition to the engineering of novel electrolyte materials, there is the opportunity to employ post-lithium chemistries. Utility of multivalent cation (Ca2+, Mg2+, Zn2+ and Al3+) transport promises a reduction in cost and increase in safety. In this review, we examine the current research focused on developing solid electrolytes using multivalent metal cation charge carriers and the outlook for their application in all solid state batteries. Keywords: all solid state battery; multivalent metal cation conductor; solid polymer electrolyte; solid inorganic electrolyte Citation: O’Donnell, L.F.; Greenbaum, S.G. Review of Multivalent Metal Ion Transport in 1. Introduction Inorganic and Solid Polymer Electrolytes. Batteries 2021, 7, 3. Within the next decade the energy needs of society are set to out pace the current https://doi.org/10.3390/ availability of low cost renewable energy sources. The lithium ion battery has been hailed batteries7010003 as the future of energy storage and is indeed an integral component of the technology sustaining our daily lives. In recent years it has become apparent that there are limits to Received: 30 November 2020 our reliance on using lithium in energy storage. Traditional organic liquid electrolytes Accepted: 28 December 2020 commonly used for Li+ transport were found to pose significant safety and environmental Published: 31 December 2020 risks due to leakage and flammability. The development of solid electrolytes in the form of solid inorganic ion conductors and solid polymer matrices is on track to improve thermal Publisher’s Note: MDPI stays neu- and mechanical stability in realization of all solid state batteries [1–3]. Unfortunately, tral with regard to jurisdictional clai- the development of improved electrolytes does not solve the problem of strained natural ms in published maps and institutio- resources [4]. Aside from possibly “running out” of mined lithium, we do not have the nal affiliations. technology nor availability of sufficient recyclable material to keep up with projected lithium demand over the next 20 years [4–6]. One way to meet the energy demands of the world in a safe, effective and environmen- Copyright: © 2020 by the authors. Li- tally sustainable way is to develop batteries using alternative charge carriers with higher 2+ 2+ 2+ 3+ censee MDPI, Basel, Switzerland. theoretical energy volume. The multivalent metal cations Ca , Mg , Zn and Al have This article is an open access article been considered to be alternatives to univalent ions since the first lithium batteries were distributed under the terms and con- under development. The challenges to using higher valence cations in solid electrolytes are ditions of the Creative Commons At- generally related to mobility loss due to high charge per volume and slow diffusion under tribution (CC BY) license (https:// ambient conditions. However, the energy payoff to employing these cations promises creativecommons.org/licenses/by/ significant increases in energy storage capacity and their availability in the Earth’s crust 4.0/). Batteries 2021, 7, 3. https://doi.org/10.3390/batteries7010003 https://www.mdpi.com/journal/batteries Batteries 2021, 7, 3 2 of 27 offer an improvement over lithium from an environmental perspective. Calcium, for exam- ple, has a theoretical energy volume of 2073 mA h cm−3, and is the fifth most abundant naturally occuring metal [7]. Magnesium has long been considered a strong candidate for beyond lithium batteries because it is the lightest of the multivalent metals, has a high volumetric charge capacity (3833 mA h cm−3) and is the eighth most abundant metal in the Earth’s crust (third most abundant element in seawater) [8]. Zinc represents an increase in volumetric charge capacity to 5851 mA h cm−3, is the 24th most abundant metal and can easily be handled under atmospheric conditions with little reactivity [9]. Aluminum, with its trivalent charge, boasts the highest volumetric charge capacity (8040 mA h cm−3) and is the most abundant metal in the Earth’s crust as well as the most recycled [10]. While we have chosen to focus on the aforementioned multivalent metal charge carriers and their transport properties in solid electrolytes, it is important to mention that there continues to be significant research in post lithium univalent cations as well. In particular, sodium and potassium technologies have emerged as promising platforms for the development of non-lithium batteries. Review of Na+ and K+ charge transport and the state of research regarding development of suitable electrolytes for them is beyond the scope of this review. However, we direct the interested reader to recent reviews covering the topics of Na+ [11–18] and K+ [19–24] technologies and the references provided therein. In this review, we explore the current knowledge of ion transport mechanisms in solid inorganic and “dry” solid polymer electrolytes that feature Ca2+, Mg2+, Zn2+ and Al3+ as their charge carriers. First, we briefly describe some of the most widely used techniques for examining ion dynamics in electrolytic systems and discuss the relevant theory behind application of these methods. This is followed by a survey of the ion transport models proposed for the respective ion conductor types. We then present findings from our review of the literature regarding what is known to date about the transport of the noted multivalent cations in all solid electrolytes. Finally, we conclude with some considerations regarding the applicability of these multivalent metal electrolytes in all solid state batteries. 2. Methods for Evaluating Ion Transport The definition of bulk conductivity in any material is described by the equation: s = ∑ miniqi (1) i where s is the conductivity, mi is the ion mobility, ni is the charge carrier ion concentration and qi is the ionic charge of the ith species. Electrical properties of a bulk material, including ionic conductivity, are often evaluated using electrochemical impedance spectroscopy (EIS), also referred to as ac impedance or dielectric spectroscopy [25]. Temperature, ion mobility and the type and volume of charge carriers all contribute to the conductive properties of the electrolyte. The general method of EIS involves application of a frequency dependent voltage signal through the sample of interest and subsequent measurement of the impedance which typically ranges from 10−2 to 108 W [26]. Conductivity measurements made as a function of temperature allow for computation of the activation energy, Ea, by least squares fitting of the conductivity to the Arrhenius equation: −Ea st(T) = A(T)exp (2) kbT where A is the pre-exponential factor, Ea is the activation energy for conduction and kb is Boltzmann’s constant. Linear variation of ionic conductivity with the inverse of absolute temperature indicates that a material obeys Arrhenius type behavior. Information regarding the type of charged species contributing to the total conduc- tivity in an electrolyte is given by measuring the transference number. Several methods have been applied to estimate transference numbers [27], though the most widely used are based on potentiostatic polarization [28–31]. In combined ac/dc polarization the sample is placed between a set of non-blocking electrodes which selectively limit transfer to the ion Batteries 2021, 7, 3 3 of 27 of interest. A constant voltage is applied to drive the cation of interest toward the anode where the cation accumulates. This creates a concentration gradient as indicated by a continuous decrease in current density until a steady state is reached [30]. The transference number can then be calculated according to: Iss(DV − I0R0) tCationx+ = (3) I0(DV − IssRss) where I0 is the initial current (t = 0), Iss is the steady state current, R0 is the initial resis- tance of the passivation layer on the electrodes before polarization, Rss is the resistance of the passivation layer after polarization and DV is the applied voltage bias [30]. While transference numbers retain their status as important contributing players in character- izing conductivity in electrolytic materials, there are several considerations that must be made when applying polarization techniques and analyzing their results. For inorganic ion conductors, the role of grain boundaries on impedance measurements becomes an important factor. It is generally accepted that the grain boundaries within a sample
Details
-
File Typepdf
-
Upload Time-
-
Content LanguagesEnglish
-
Upload UserAnonymous/Not logged-in
-
File Pages27 Page
-
File Size-