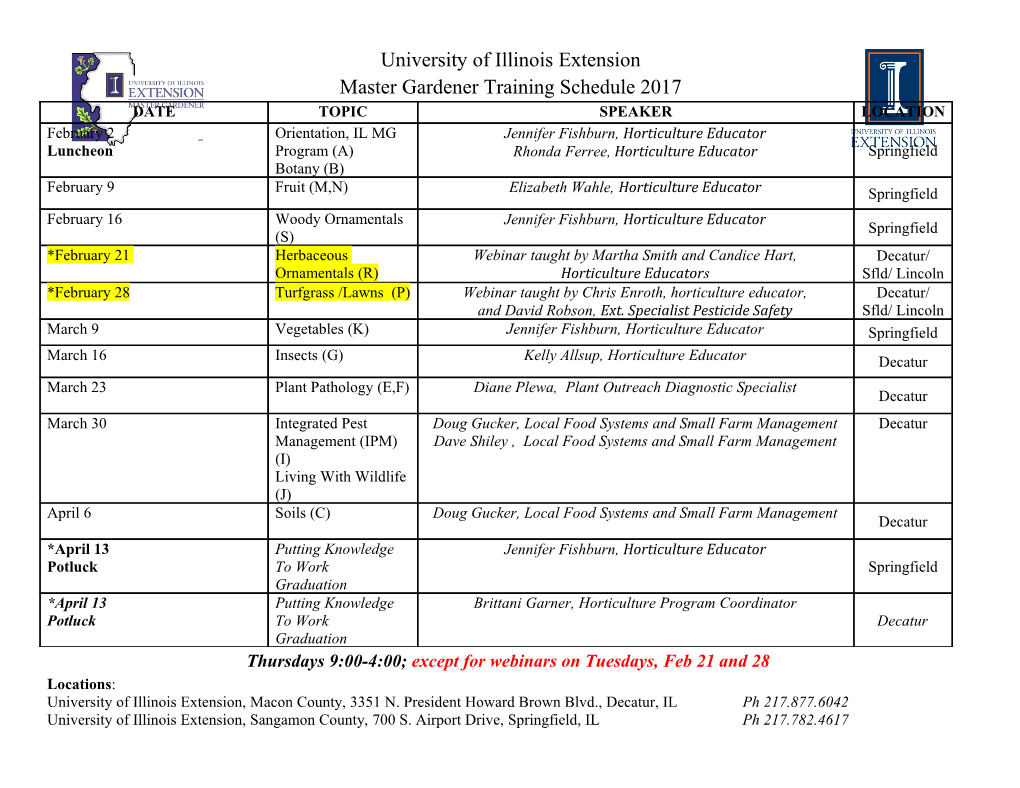
1 VIRAL FACTORS IN INFLUENZA PANDEMIC RISK ASSESSMENT 2 Marc Lipsitch*, Center for Communicable Disease Dynamics, Departments of 3 Epidemiology and Immunology and Infectious Diseases, Harvard T. H. Chan School of 4 Public Health, Boston, MA, USA 5 Wendy Barclay, Division of Infectious Disease, Faculty of Medicine, Imperial College, 6 London, UK 7 Rahul Raman, Department of Biological Engineering, Koch Institute for Integrative 8 Cancer Research, Massachusetts Institute of Technology, 77 Massachusetts Avenue, 9 Cambridge MA 02139 USA 10 Charles J. Russell, Department of Infectious Diseases, St. Jude Children’s Research 11 Hospital, 262 Danny Thomas Place, Memphis, TN 38105-3678, USA 12 Jessica A. Belser, Centers for Disease Control and Prevention, Atlanta, GA, USA 13 Sarah Cobey, Department of Ecology and Evolutionary Biology, University of Chicago, 14 Chicago, IL, USA 15 Peter Kasson, Departments of Biomedical Engineering and Molecular Physiology and 16 Biological Physics, University of Virginia, Charlottesville, VA, USA 17 James O. Lloyd-Smith, Department of Ecology and Evolutionary Biology, University of 18 California at Los Angeles, CA, USA and Fogarty International Center, National Institutes 19 of Health, Bethesda, MD, USA 1 20 Sebastian Maurer-Stroh, Bioinformatics Institute, Agency for Science Technology and 21 Research, Singapore, Singapore; National Public Health Laboratory, Communicable 22 Diseases Division, Ministry of Health, Singapore, Singapore; School of Biological 23 Sciences, Nanyang Technological University, Singapore, Singapore 24 Steven Riley, MRC Centre for Outbreak Analysis and Modelling, Department of 25 Infectious Disease Epidemiology, School of Public HealthImperial College London 26 Catherine Beauchemin, Department of Physics, Ryerson Unversity, Toronto, Canada 27 Trevor Bedford, Vaccine and Infectious Disease Division, Fred Hutchinson Cancer 28 Research Center, Seattle, WA, USA 29 Thomas C Friedrich, Department of Pathobiological Sciences, University of Wisconsin 30 School of Veterinary Medicine, Madison, WI, USA 31 Andreas Handel, Department of Epidemiology and Biostatistics, College of Public Health, 32 University of Georgia, Athens, GA, USA 33 Sander Herfst, Department of Viroscience, Erasmus Medical Center, Rotterdam, NL 34 Pablo R. Murcia, MRC-University of Glasgow Centre for Virus Research, Glasgow, UK 35 Benjamin Roche, IRD/UPMC UMMISCO, Montpellier, France 36 Claus O. Wilke, Center for Computational Biology and Bioinformatics, Institute for 37 Cellular and Molecular Biology, and Department of Integrative Biology. The University of 38 Texas at Austin. Austin, TX 78712 Street address: 2500 Speedway, A4800. 2 39 Colin Russell, Department of Veterinary Medicine, University of Cambridge, Madingley 40 Road, Cambridge, CB3 0ES, UK 41 42 43 44 *To whom correspondence should be addressed. Department of Epidemiology, Harvard 45 T.H. Chan School of Public Health, 677 Huntington Avenue, Boston, MA 02115 USA. 46 [email protected] 47 3 48 ABSTRACT 49 The threat of an influenza A virus pandemic stems from continual virus spillovers from 50 reservoir species, a tiny fraction of which spark sustained transmission in humans. To 51 date, no pandemic emergence of a new influenza strain has been preceded by detection 52 of a closely related precursor in an animal or human. Nonetheless, influenza surveillance 53 efforts are expanding, prompting a need for tools to assess the pandemic risk posed by 54 a detected virus. The goal would be to use genetic sequence and/or biological assays of 55 viral traits to identify those non-human influenza viruses with the greatest risk of 56 evolving into pandemic threats, and/or to understand drivers of such evolution, to 57 prioritize pandemic prevention or response measures. We describe such efforts, identify 58 progress and ongoing challenges, and discuss three specific traits of influenza viruses 59 (hemagglutinin receptor binding specificity, hemagglutinin pH of activation, and 60 polymerase complex efficiency) that contribute to pandemic risk. 4 61 INTRODUCTION 62 Aquatic birds are the main reservoir of influenza A viruses in nature (1). Influenza viruses 63 from aquatic birds sporadically enter terrestrial bird and mammalian host populations 64 and achieve sustained circulation in these new hosts (2), sometimes after reassortment 65 with influenza viruses already circulating in the new host (3). Adaptation of viruses from 66 aquatic birds to mammals involves a change in tissue tropism from intestinal to 67 respiratory epithelia (4, 5). 68 Multiple influenza A subtypes—defined by the patterns of antibody recognition of two 69 surface proteins, hemagglutinin (HA) and neuraminidase (NA)—circulate in avian species 70 and swine at any given time. Among these, a number are known to cause sporadic 71 zoonotic infections in humans (6). Hundreds of human infections with avian influenza 72 viruses were detected in the last decade, for example H5N1 and H7N9 (7) as well as 73 swine influenza viruses, e.g. an H3N2 variant that spilled over into humans attending 74 agricultural shows in the early 2010s, H3N2v (8). In addition, zoonotic infections with 75 other viruses from poultry have occurred, including for example H7N7 (9), H10N8 (10), 76 H6N1 (11), H9N2 (12), and H5N6 (13); for more examples and a fuller discussion see 77 (14). The severity of zoonotic influenza A infections ranges from clinically inapparent 78 (15, 16) to fatal (17, 18). 79 Although secondary transmission can occur following some of these spillover events 80 (19), only a very small proportion of them—four in the last hundred years, which seems 81 to be close to the historical average (20)—led to sustained person-to-person 5 82 transmission with global spread (Box 1). There are 18 known HA types and 11 known NA 83 types (21), which could theoretically be found in any combination. So far, sustained 84 spread in humans has been limited to the H1N1, H2N2, and H3N2 subtypes (22), though 85 it is possible that other subtypes circulated prior to 1918, the year of the first pandemic 86 from which viruses are available for study (23). Multiple virus–host interactions are 87 necessary for replication and onward transmission; the differences in the genetic 88 requirements to accomplish each of these interactions in humans versus other animals 89 provide a barrier to sustained transmission following spillover (24). 90 Experiments in ferrets have been used to measure viral transmissibility via respiratory 91 droplets (in this review we use this term to refer to any transmission through the air 92 between ferrets that are not in direct or indirect physical contact). Droplet transmission 93 in ferrets is a useful, albeit imperfect, correlate of the potential of influenza strains to 94 transmit efficiently in human populations (25). For this reason, some have argued that 95 there is a general phenotype of "transmissibility by respiratory droplets in mammals" 96 such that experiments to select for such transmission in droplets in ferrets are 97 important models of the process of adaptation to human transmission (26, 27). This 98 view is not universally shared (28). Starting from an zoonotic highly pathogenic avian 99 influenza isolate from a human case of infection (or a reassortant of the HA from a 100 different zoonotic H5N1 highly pathogenic avian influenza isolate, with the other 101 segments from the 2009 pandemic H1N1 strain), it was shown that certain specific traits 102 that had been previously associated with mammalian host adaptation were required to 103 achieve respiratory droplet transmission. These ferret-transmission phenotypes in turn 6 104 were associated with certain genetic changes relative to the original avian viruses (26, 105 27, 29). These specific changes occur both in HA and in polymerase-complex proteins. 106 The rationale for these experiments was that, because the ferret model recapitulates 107 many features of human infection, changes identified in adaptation to ferret 108 transmission would also be important for adaptation to sustained transmission in 109 humans (30, 31), though this can never be known with certainty (28). Notably, viruses 110 isolated from humans who were infected by contact with birds show some of these 111 changes (32, 33), particularly the change at amino acid 627 of the PB2 gene (34-36), 112 which often affects polymerase complex efficiency (see below). This indicates that even 113 the first generation of human infection from nonhuman hosts can initiate a process of 114 host adaptation. It also indicates that not all the human-adaptive changes must be in 115 place in the avian reservoir to initiate this process. Some human infections, including 116 some zoonotic cases (17, 35, 37, 38) and some cases early in a pandemic (39-43), 117 involve viruses that are not yet fully human-adapted (see below and Tables 2–4). The 118 interpretation of some of these isolates is complicated by uncertainty about whether 119 they were passaged in hen’s eggs at some point in their history. 120 Certain types of countermeasures against an influenza pandemic are effective only 121 against one lineage of viruses – for example, creating stockpiles or seed stocks of 122 vaccines against a particular subtype, or culling poultry infected with that subtype. It is 123 not currently feasible to invest in such countermeasures against all viruses circulating in 124 avian or other reservoirs, or even against all those causing known zoonotic cases. 7 125 Therefore, there would be value in an accurate system to assess the relative pandemic 126 risks posed by each virus and prioritize them for the development of such strain-specific 127 countermeasures, while directing fewer resources to strains of lower concern (44). This 128 consideration has motivated calls for comprehensive analysis of all available data to 129 assess the threat to public health posed by these strains. One response is the CDC’s 130 Influenza Risk Assessment Tool (IRAT) (45), which incorporates elements including 131 properties of the virus, field and epidemiological findings, and attributes of the human 132 population to provide a framework to differentiate among novel influenza viruses 133 thought to possess pandemic potential.
Details
-
File Typepdf
-
Upload Time-
-
Content LanguagesEnglish
-
Upload UserAnonymous/Not logged-in
-
File Pages96 Page
-
File Size-