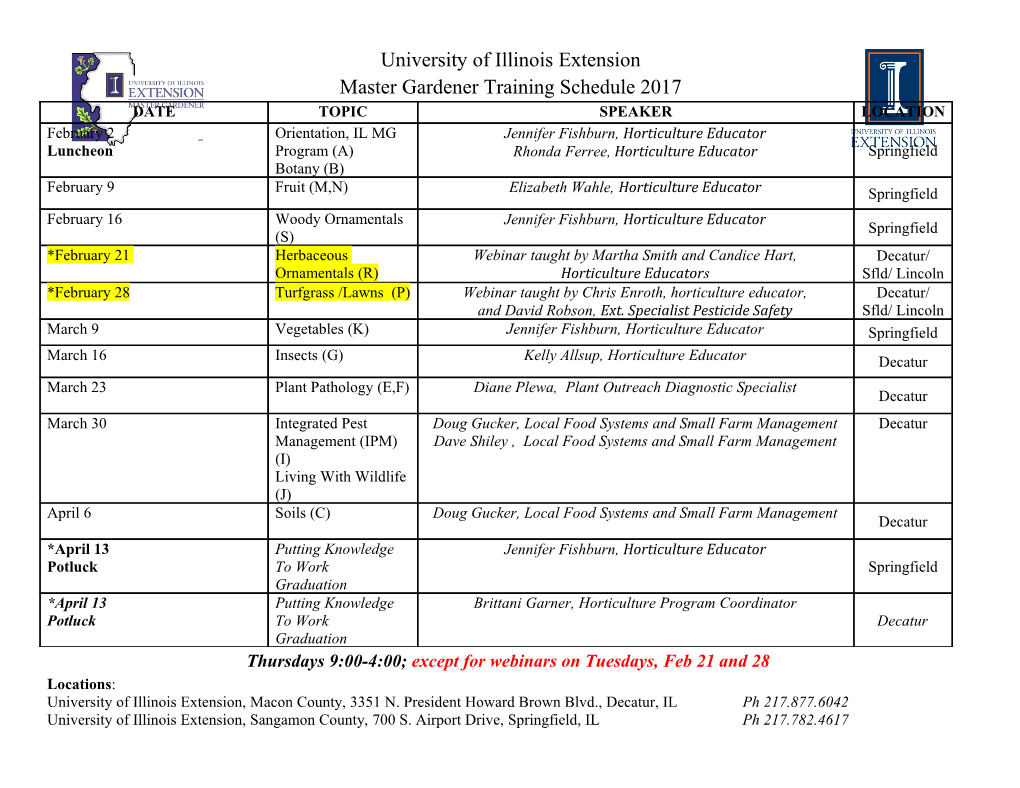
SERI!TP-281-1846 SOLARTHERMAL CONVERSION Frank Kreith Richard T. Meyer November 1982 Prepared under Task No. 1430.76 WPA No. 153C-82 SOLAR ENERGY RESEARCH INSTITUTE A Division of Midwest Research Institute 1617 Cole Boulevard Golden, Colorado 80401 Prepared for the U.S. DEPARTMENT OF ENERGY Contract No. EG-77-C-01-4042 SOLAR THERMAL CONVERSION* TITLE ABSTRACT High temperature applications of solar energy for electricity and industrial process heat are being attained with solar power towers. *This article has been prepared for submission as an invited paper to American Scientist, which is published by Sigma Xi, The Scientific Research Society. Frank Kreith is currently a Sigma Xi lecturer. AUTHOR DESCRIPTIONS Frank Kreith is the Chief of the Solar Thermal Research Branch at the Solar Energy Research Institute (SERI). An internationally renown researcher in the thermal sciences and a pioneer in the solar energy field, Dr. Kreith was a member of the original team that established SERI in Colorado. Prior to that he was president of the engineering consulting firm ECS and Professor of Engineering and Chairman of the Council for Research and Creative Activities at the University of Colorado. He is also the Technical Editor of the ASME Transactions Journal of Solar Energy Engineering. Richard T. Meyer is the Solar Thermal Science Writer with the SERI Technical Information Branch. He received his B.s. and Ph.D. in physical chemistry from the University of Wisconsin and the University of California at Berkeley, respectively. He has previously been engaged in physical/materials science research at Sandia National Laboratories and as president and senior energy technologist for Western Energy Planners, Ltd. SOLAR THERMAL CONVERSION Energy is not a .good unto itself; it is valued rather as a means of satisfying important needs of a society. In classical thermodynamics, energy is defined as the capacity to do work; but from a more practical point of view, energy is the main stay of any industrial society. In the United States, energy is currently provided by seven primary sources: petroleum, natural gas, coal, hydro-power, nuclear fission, geothermal, and wood and waste. The first three of these sources are fossil fuels. They are stored forms of solar energy that received their solar input eons ago, have changed their characteristics over time, and now are in a highly concentrated and convenient form. It is apparent, however, that these stored forms of solar energy are being used so rapidly that they soon will be depleted. To maintain our present social structure, it is desirable, therefore, that we supply an increasing portion of our energy needs from renewable sources. The radiative solar energy reaching the earth during each month is approximately equiva­ lent to the entire world supply of fossil fuels. Thus, from a purely thermodynamic point of view, the global potential of solar energy is many times larger than the current energy use. However, many technical and economic problems must be solved before large-scale use of solar energy can occur. The future of solar power deployment depends on how we deal with these constraints, which include scientific and technological problems, marketing and financial limitations, and political and legislative actions including equitable taxation of renewable energy sources. Approximately 30 percent of the solar energy impinging on the earth is reflected back into space. The remaining 70 percent, approximately 120,000 terawatts [l terawatt is equal to 1012 watts], is absorbed by the earth and its atmosphere. Solar radiation reaching the earth consists of the beam radiation that casts a shadow and can be con­ centrated and the diffuse radiation that has been scattered along its path in space from sun to earth. The solar radiation reaching the earth degrades in several ways. Some of the radiation is directly absorbed as heat by the atmosphere, the ocean, and the ground. Another component produces atmospheric and oceanic circulation. A third component evaporates, circulates, and precipitates water in the hydrologic cycle. Finally, a very small fraction is captured by green plants and drives the photosynthetic process. 1 For solar energy to be used in meeting the demands of a society, it must be converted into heat, mechanical power, or electricity. The conversion methods can be divided into natural and technological conversion systems (see Figure O. In natural conversion, the biosphere, i.e, earth, wind or water, serves as a solar energy collector and storage. Since no man-made collectors are needed, the cost of energy from natural systems is largely determined by the conversion equipment, such as a wind turbine. In technological con­ version systems, solar energy must be absorbed by man-made structures or collectors; the amount of insolation intercepted is determined by the total area and orientation of the collecting surface at a given geographic location (Kreith and Kreider 1978). The source of the sun's energy is a hydrogen-to-helium thermonuclear reaction. The outer layer of the sun, from which the solar radiation emanates, has an equivalent black body temperature of about 5760 K (5487° C). The solar energy reaching the earth, called insolation, is in the form of photons, or radiation, covering a range of wavelengths cor­ responding approximately to a 5760 K black body. To convert this radiation into useful energy, one may either use photons in the appropriate wavelength range of the spectrum to generate electricity directly by photovoltaic conversion devices; or one may use-the thermal part of the radiation spectrum to heat a working fluid by thermal conversion in a solar collector. The following discussion is concerned only with solar thermal conversion systems. The thermal conversion process of solar energy is based on well-known phenomena of heat transfer (Kreith 1976). In all thermal conversion processes, solar radiation is absorbed at the surface of a receiver, which contains oris in contact with flow passages through which a working fluid passes. As the receiver heats up, heat is transferred to the working fluid which may be air, water, oil, or a molten salt. The upper temperature that can be achieved in solar thermal conversion depends on the insolation, the degree to which the sunlight is concentrated, and the measures taken to reduce heat losses from the working fluid. Since the temperature level of the working fluid can be controlled by the velocity at which it is circulated, it is possible to match solar energy to the load · requirements, not only according to the amount but also according to the tempera.ture level, Le., the quality of the energy required. In this manner, it is possible to design con­ version systems that are optimized according to both the first and the second laws of thermodynamics. 2 The collection and conversion of the solar radiation to thermal energy depends on the collector design and the relative amounts of direct beam and diffuse radiation absorbed by the collector (Kreider and Kreith 1981). As indicated in the following discussion of solar thermal collectors, the collectors used for higher temperature applications can col­ lect only the direct radiation from the sun. Figure 2 shows the annual average daily direct normal solar radiation for the contiguous United States, Alaska, and Hawaii; values range from under 2.78 kW/hr/m2 (10 MJ/m2) to over 7.22 kW/hr/m2 (26 MJ/m2) (Solar Energy Research Institute 1981). Peak direct solar radiation at noon during a clear day averages about I kW/m2• Generally speaking, the southwestern and western regions of the country receive direct normal solar radiation levels sufficiently high for most high temperature solar thermal conversion applications. High temperature heat is needed by industry for process heat and by utilities for elec­ tricity. In 1980, the last year for which statistics are available, industry and utilities accounted for approximately 73 percent of the 76.3 quads of energy consumed in the United States (Energy Information Administration 1980). The industrial process heat por­ tion alone was 20.6 quads (17 percent). Figure 3 displays a recent analysis by the Solar Energy Research Institute (Krawiec et al 1981) of the distribution of industrial process heat requirements by process temperature. It can be seen that 48.9 percent of the pro­ cess heat total falls below 500°F (260° C) and 34.0 percent is above 10aaoF (538° C). 3 SOLAR THERMAL COLLECTORS To be economically worthwhile, a solar collector must be selected to meet the needs of a given task. This is called end-use matching. From the time of solar architecture in ancient Greece over 2,500 years ago, many types of solar collectors have been developed. Several different collector types currently are in use for various applications, energy loads, and temperatures. These collector types include solar pond, flat-plate, evacuated-tube, line-focus," point-focus, and central receiver. Figure 4 shows the approximate operating temperature ranges for the collector types (Kutscher et ale 1982); brief descriptions follow. Solar ponds are bodies of water that can simultaneously absorb and store solar energy. There are essentially two types: shallow ponds and salt gradient ponds. Shallow ponds consist of bags of water which are heated by the sun during the day and must be drained at night. Their upper level of temperature capability is 120° F (49°C), and their thermal efficiency is relatively low. Alternatively, salt gradient ponds can supply heat at temperatures up to 180° F (82° C) in favorable locations. The salt gradient pond consists of three "layers." The bottom or storage layer consists of a uniform high concentration salt water solution, while the top layer consists of a uniform salt water solution of low concentration. Between these two layers is the gradient layer in which the concentration increases with distance from the top.
Details
-
File Typepdf
-
Upload Time-
-
Content LanguagesEnglish
-
Upload UserAnonymous/Not logged-in
-
File Pages31 Page
-
File Size-