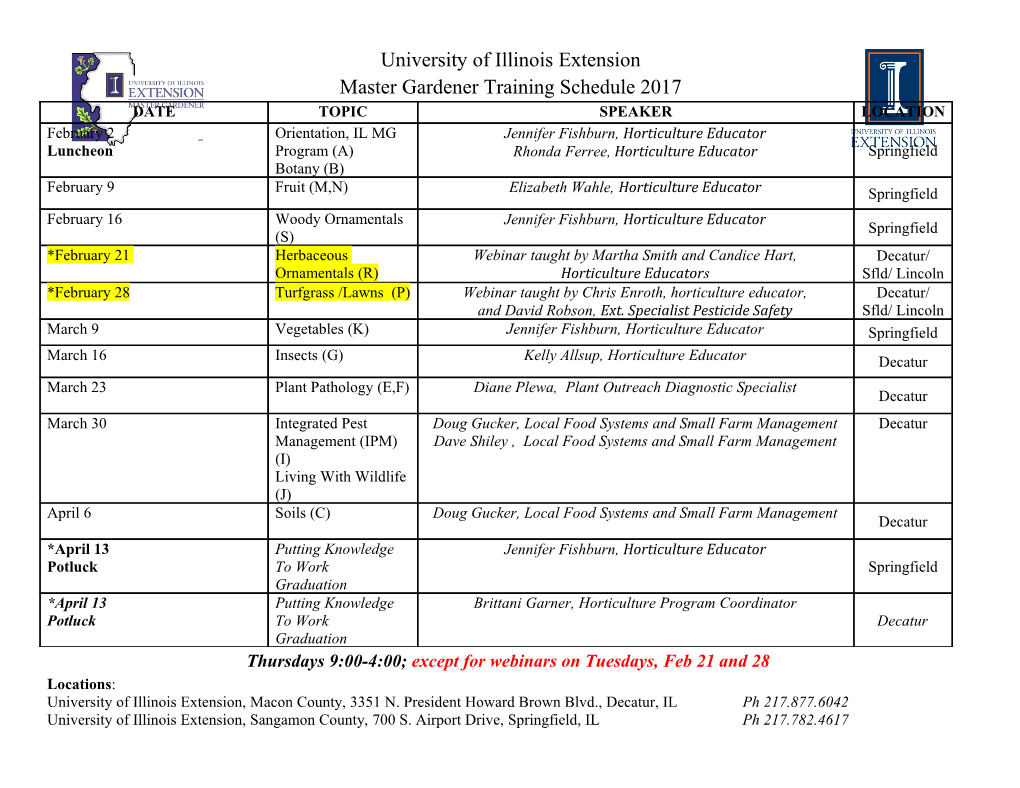
J. Rothberg October 12, 2001 Introduction to Quantum Mechanics – part 5 1 Nuclear Magnetic Resonance 1.1 Relaxation Times The Nuclear Magnetic Resonance setup includes a large static magnetic field, B0 in the z direction. The magnitude of this field is typically between 1 and 2 Tesla (10,000 to 20,000 gauss). For medical applications when large volumes and high fields are needed the field is produced with a superconducting solenoid. There are additional coils which modify this field to achieve spatial maps. The sample of nuclei with spin usually consists of protons in water or organic materials but also may include 19Fluorine, 13Carbon or other non-zero spin nuclei. 1.1.1 Spin-Lattice Relaxation - T1 When the B0 magnetic field is established a fraction of the protons go into the lower energy state with the magnetic moment aligned with the magnetic field. This is a thermal relaxation process depending on interactions and energy exchange with the medium. The fraction of spins that is aligned is only about one in a million at room temperature at 1 Tesla but this still provides about 1017 aligned spins in a typical sample. An important question is how long it takes to establish this spin alignment. The characteristic time for spins to align with the magnetic field is called T1 or the “Spin- Lattice” or the “Longitudinal” relaxation time or “energy” relaxation time. The growth of spin alignment or longitudinal magnetization follows an exponential of the form (1−exp−t/T1 ). Typical values for T1 in biological tissue range from 200 ms to two seconds. If we were to force the spins to be aligned in the higher energy state (magnetic moment opposite the B0 field) or in another direction then the spins would relax back to the lower energy state with this characteristic time. The spin directions can be controlled with appropriate external applied magnetic fields as will be described but the T1 relaxation process is always present. 1.1.2 Spin-Spin Relaxation - T2 If we were to align the spins in the x, y plane (perpendicular to B0) using applied B1 magnetic fields the collection of spins will start to precess about B0, the z direction. This precession of the magnetic moments can be detected with a pickup coil in the x, y plane. As a consequence of encounters with local magnetic fields due to other molecules in the medium their precession 17 frequency about B0 is modified and the (≈ 10 ) spins in the sample begin to get out of phase as they precess. This results in a reduction of the strength of the magnetization and a reduced signal in the pickup coil. The characteristic time for this loss of magnetization is called the “Spin-Spin” relaxation time or T2. This characteristic time depends on the nature of the material and is of order 40 ms to 200 ms in biological tissue at 1 T. 1 0 1.1.3 Field Non-uniformity - T2 An additional important mechanism for loss of magetization is due to inhomogeneities in the B0 magnetic field. Since the proton sample has some extention in space, protons in different parts of the sample will reside in regions with slightly different values of B0 and therefore precess at slightly different frequencies. This results in a further loss of signal size. ∗ The reduction in net magnetization due to these effects has a characteristic time T2 . Normally the field inhomogeneities ∆B0, dominate the reduction of magnetization and signal. 1 1 1 ∗ = 0 + T2 T2 T2 0 where T2 is the dephasing time due to B0 variations across the sample volume. 1.2 Pulsed NMR The large static magnetic field B0, is in the z direction. The magnetization will precess about B0 with angular frequency (Larmor Frequency) ω0 = µ B0. 2 We can apply short pulses of the B1 (rotating) oscillating field which lies in the x, y plane. If the B1 field oscillates with the precession frequency ω0, then the system will have a simple behavior as described in the rotating frame. ◦ 180 pulse If the duration and strength of the B1 pulse are sufficient for the spins originally ◦ lined up with B0 to rotate through 180 around a line in the x, y plane then the magnetization ◦ will point in the direction opposite B0. The duration of such a 180 or “π” pulse or “inversion” pulse is tπ = π/(γB1) where γ = ω0/B0. If B1 is large the the duration can be short; this is called a “hard” pulse. Conversely if B1 is small then a wide pulse is needed: a “soft” pulse. Following this 180◦ pulse the magnetization will undergo a longitudinal relaxation with char- acteristic time T1 back to the lowest energy state along B0. ◦ 90 pulse If we apply a B1 pulse of appropriate duration and amplitude the magnetization ◦ π will precess 90 from z into the x, y plane. Following this 2 pulse the magnetization will precess about the z axis and at the same time will relax back to the B0 field direction. During the precession about z dephasing reduces the magnetization as a consequence of spin-spin interactions and B0 field inhomogeneities. This situation is called “Free Induction Decay”, FID, and the signal appears in the pickup coil as an oscillation with decreasing amplitude. 3 A pickup coil in the x, y plane will detect an induced signal as the magnetization precesses around the z axis. The dephasing will cause a drop in the amplitude of the observed signal. 180◦ pulse followed by a 90◦ pulse If a 90◦ pulse is applied some time after the 180◦ pulse then the precession signal can be detected in the x, y plane and the signal amplitude ◦ can be used to determine the T1 relaxation time. A 90 pulse can be applied at various delay times following a 180◦ pulse to allow one to trace out the longitudinal relaxation curve. ◦ ∗ Notice the rapid decay of magnetization after the 90 pulse due to the T2 relaxation. 4 Spin Echo The dephasing in the x, y plane is due to very different mechanisms: a) the random spin-spin interactions with local magnetic fields in the material; b) the dephasing due to B0 non-uniformity depends on the location of the magnetic moment in the material. The B0 non-uniformity is not time dependent (over short time scales). The portion of the dephasing due to the non-uniform field can be recovered using a very elegant trick, Spin Echo. One waits until the dephasing has taken place and then one applies a 180◦ pulse. This pulse flips all the spins in the x, y plane and then they continue to precess about z. But now the spins, previously out of phase, recover their coherence. This recovery of magentization only applies to the losses due to the B0 field non-uniformity. The magnetization loss due to spin-spin interactions is random and is not recovered by the precession reversal. 5 This situation resembles a race with contestants running at different speeds who therefore get out of phase. If at some moment each runner reverses direction then they will be in phase again when they get back to the starting line. This assumes that their speeds after reversal are the same as before. This is a model for the effect of recovery of phase coherence in the non-uniform B0 field. The non-uniformities are the same after reversal as before so a reversed direction of precession brings the magnetization vectors back in phase. sequence of 180◦ pulses After each pulse the signal amplitude grows as the phase co- herence is recovered and then declines again due to dephasing. The maximum amplitude of the rephased signals decreases with time constant T2, due to the non-recoverable dephasing from random interactions in the medium. In medical NMR (Magnetic Resonance Imaging, MRI) different values of T1 and T2 are associated with different biological tissues. Complex pulse sequences permit a determination of T1 and T2 in different parts of the sample volume and provide images of internal organs. 6 7.
Details
-
File Typepdf
-
Upload Time-
-
Content LanguagesEnglish
-
Upload UserAnonymous/Not logged-in
-
File Pages7 Page
-
File Size-