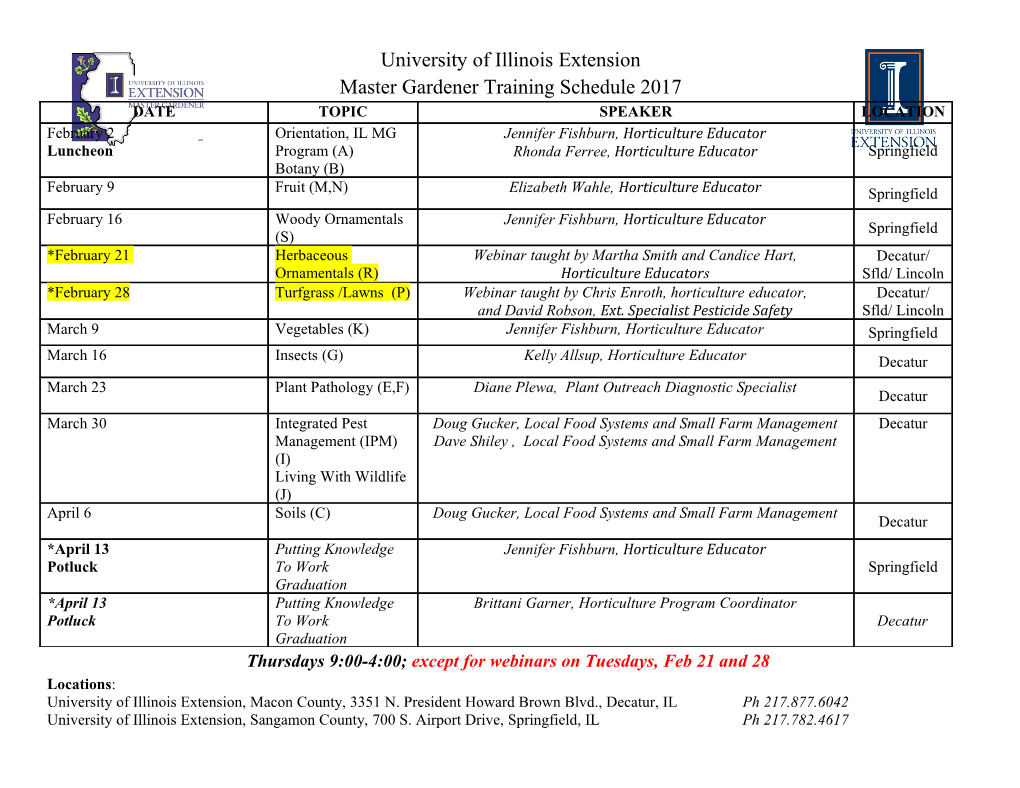
Bioscience Reports, Vol. 25, Nos. 1/2, February/April 2005 (Ó 2005) DOI: 10.1007/s10540-005-2848-y Protein Arrays in Functional Genome Research Christian Maercker1 Whole-genome analyses become more and more necessary for pharmaceutical research. DNA chip hybridizations are an important tool for monitoring gene expression profiles during diseases or medical treatment. However, drug target identification and validation as well as an increasing number of antibodies and other polypeptides tested as potential drugs produce an increasing demand for genome-wide functional assays. Protein arrays are an important step into this direction. Peptide arrays and protein expression libraries are useful for the identification of antibodies and for epitope mapping. Antibody arrays allow protein quantification, protein binding studies, and protein phosphorylation assays. Tissue micro- arrays give a detailed information about the localization of macromolecules. More complex interactions can be addressed in cells spotted in array format. Finally, microfluidics chips enable us to describe the communication between cells in a tissue. In this review, possibil- ities, limitations and chances of different protein array techniques are discussed. KEY WORDS: Protein chips; antibody chips; peptide arrays; microfluidics; functional genome analysis; protein binding; protein phosphorylation. ABBREVIATIONS: CGH: comparative genomic hybridization; CE-IVD: a certified product for in vitro diagnostics according to CE directive of the EU (‘‘Communautes Europe´ennes’’); ChIP: chromatin immunoprecipitation; GFP: green fluorescent protein; MEA: microelectrode arrays; RNAi: RNA interference; SNP: single nucleotide polymor- phism. INTRODUCTION High-throughput assays are indispensible for comprehensive functional genome re- search. The development of these techniques has been promoted again by the suc- cessful completion of the human genome sequencing project (Mc Pherson et al., 2001; Venter et al., 2001). This result, which was made possible by automated sequencing techniques and enormous progress in bioinformatics, is an important prerequisite for the application of whole-genome screens. The insertion of all sequence information into databases allows the design of comprehensive cDNA and oligonucleotide-based gene chips. DNA arrays are the state-of-the-art tool for gene expression analyses (Brown and Botstein, 1999), but also for the detection of chromosomal aberrations by comparative genomic hybridization on DNA microarrays (matrix-CGH; for review see Lichter et al., 1RZPD German Resource Center for Genome Research GmbH, Berlin-Heidelberg, Im Neuenheimer Feld 580, D-69120, Heidelberg, Germany. E-mail: [email protected] 57 0144-8463/05/0400-0057/0 Ó 2005 Springer Science+Business Media, Inc. 58 Christian Maercker 2000), identification of protein binding DNA stretches (chromatin immunoprecipi- tation (ChIP); Cawley et al., 2004), and single nucleotide polymorphisms (SNPs; Woods et al., 2004). DNA–DNA and DNA–RNA hybridizations are established methods, which allow the detection of each single gene and its splice-variants. Not only RNA profiles are relevant for diagnostics, but also the genetic predisposition, which can be analyzed by hybridizing SNP chips or sequencing arrays. Very recently, Roche Diagnostics has launched the first pharmacogenetic gene chip with CE-IVD label. The AmpliChip CYP450 analyzes genes of the cytochrome P450 system involved in the metabolism of many different drugs. Metabolization rates of individual drugs can be calculated. Therefore, this tool helps to select certain drugs in appropriate concentrations for individual patients. RNA interference (RNAi) gene knock-downs and other genome-wide screens in pharmaceutical research are accompanied by gene expression profiles (for review see Carpenter and Sabatini, 2004). However, information about the expression level is not sufficient to describe gene function. This is not only due to the weak correlation between transcription activity and protein concentration (Gygi et al., 1999). Post-translational modifica- tions cannot be recognized at the RNA level. For example, signal transduction processes in the cell are predominantly determined by phosphorylation steps. Also binding partners of proteins, localization of proteins in cellular compartments, enzymatic reactions, and many other functions are not appropriate to be analyzed by RNA expression analysis. Therefore, proteomics now plays a pivotal role in genome research. However, different from DNA- or RNA-based hybridization conditions, which are nearly the same for all of the genes, highly parallelized assays with proteins are very difficult to establish. Solubility, pH optima, and buffer conditions are very specific for each molecule. Different techniques are applied to investigate as many proteins in parallel as possible. 2D-gel electrophoresis allows the separation of thousands of proteins within the same experiment. Mass spectrometry methods (e.g., MALDI-TOF, SELDI) have evolved into very accurate, sensitive, and quick tools, which allow the extraction of many small spots from the gel and the analysis in a high-throughput manner. Database searches with those results make it possible to estimate peptide sequences and protein modifications (Wiesner and Bougmi, 2002; for review see Sickmann et al., 2003). Moreover, mass spectrometry can help to identify protein-protein interactions (Schulze and Mann, 2004). This review gives an overview about the state-of-the-art in protein chip technology. Polypeptides are immobilized on a matrix and are assayed individ- ually in parallel in a single experiment. Peptides, polypeptides, cell lysates, whole cells, or tissue samples are suitable as probes. Applications for different arrays are discussed. PEPTIDE ARRAYS Ronald Frank’s group has developed a method which allows to immobilize up to 8.000 peptides on cellulose membranes (Frank, 2002). Recently, they also have started to use glass as matrix material. Step by step, activated amino acids are pipetted onto defined positions and built up to peptides. After each pipetting step, Protein Arrays in Functional Genome Research 59 a washing procedure removes excess of amino acids and chemicals to ensure the purity of the peptide library. The spotted molecules have a length of at most 30 amino acids with a concentration between 1 and 5 nmol. The peptides allow to detect interactions between enzymes and substrates, between antibodies and anti- genes, between receptors and hormones, and between nucleic acids and tran- scription factors. Even modifications of the macromolecules on the membrane are possible. In combination with compound libraries it is possible to detect and identify new active drug lead molecules. In further steps, these substances can be brought into solution and used for functional studies. An example is the coupling of the N-terminus of a peptide with a fluorescent dye to estimate the specificity of proteases. Together with kinases and radioactively labeled ATP the peptides can be tested as kinase substrates. By compatible peptide chemistry, drug candidates can be changed into active agents. Frank Breitling and collegues developed a very flexible method for the synthesis of peptides on membranes in a high density with the help of a classical laser printer (Bischoff et al., 2002; Breitling et al., 2002). The coloured toner particles are substituted by amino acid-toner particles, which consist of a ‘‘solid’’ solvent (diphenylformamide) and the amino acid. After a temperature increase, the amino acids are specifically released and coupled to the membrane or to the already syn- thesized peptide. This technique allows to build up peptides with lengths varying between 15 and 20 amino acids (Fig. 1). The low spreading caused by adjusting of the solvent by selective heating and high speed of the printer allows complexities of millions of different peptides on a single chip. This makes the arrays appropriate for the presentation of overlapping peptide sequences, which is applicable for the antigene mapping of autoreactive or infection related antibodies. In addition to the diagnostic aspect, libraries with different L- and D-peptides can be produced. D-peptides consist of D-amino acids, which are hardly degraded, because they do not occur in nature. Those molecules are particularly applicable for the development of new therapeutics. Fig. 1. Amino acid (isoleucine) printed on glass surface by peptide laser printer (400 spots/cm2). Kindly provided by Frank Breitling, German Cancer Research Center, Heidelberg. 60 Christian Maercker Fig. 2. (a, b). Different coloured toner particles were coupled to pixel electrodes on a chip. Bar: 80 lm (Courtesy of Frank Breitling, F. Ralf Bischoff, Volker Stadler, German Cancer Research Center, Heidelberg). Novel chips, developed by the same group, are covered with pixel electrodes, which facilitate an accurate positioning of molecules. First trials with different col- ored toner particles have already been successful (Fig. 2). In ongoing experiments, particles containing derivatives of modified amino acids are transferred to the same chips with a modified surface chemistry (Bischoff et al., 2004). PROTEIN ARRAYS Peptides are not appropriate for functional assays, because they only represent a small fraction of a whole protein, for example a protein kinase. For such assays complete proteins have to be immobilized to be able to detect enzymatic activities on a specific spot. Different publications showed that it is possible to produce specific proteins in bacteria,
Details
-
File Typepdf
-
Upload Time-
-
Content LanguagesEnglish
-
Upload UserAnonymous/Not logged-in
-
File Pages14 Page
-
File Size-