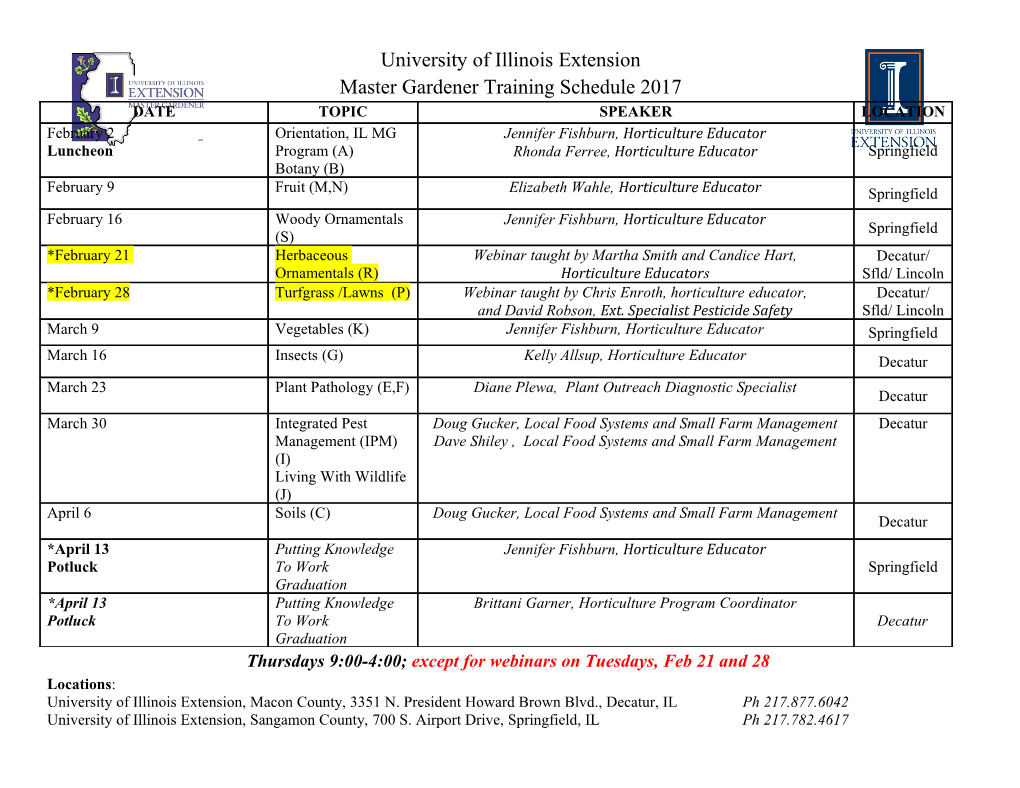
This is a repository copy of Rapid accelerations of Antarctic Peninsula outlet glaciers driven by surface melt. White Rose Research Online URL for this paper: http://eprints.whiterose.ac.uk/150042/ Version: Published Version Article: Tuckett, P.A., Ely, J.C. orcid.org/0000-0003-4007-1500, Sole, A.J. et al. (4 more authors) (2019) Rapid accelerations of Antarctic Peninsula outlet glaciers driven by surface melt. Nature Communications, 10. ISSN 2041-1723 https://doi.org/10.1038/s41467-019-12039-2 Reuse This article is distributed under the terms of the Creative Commons Attribution (CC BY) licence. This licence allows you to distribute, remix, tweak, and build upon the work, even commercially, as long as you credit the authors for the original work. More information and the full terms of the licence here: https://creativecommons.org/licenses/ Takedown If you consider content in White Rose Research Online to be in breach of UK law, please notify us by emailing [email protected] including the URL of the record and the reason for the withdrawal request. [email protected] https://eprints.whiterose.ac.uk/ ARTICLE https://doi.org/10.1038/s41467-019-12039-2 OPEN Rapid accelerations of Antarctic Peninsula outlet glaciers driven by surface melt Peter A. Tuckett1, Jeremy C. Ely 1*, Andrew J. Sole 1, Stephen J. Livingstone 1, Benjamin J. Davison2, J. Melchior van Wessem3 & Joshua Howard1 Atmospheric warming is increasing surface melting across the Antarctic Peninsula, with unknown impacts upon glacier dynamics at the ice-bed interface. Using high-resolution 1234567890():,; satellite-derived ice velocity data, optical satellite imagery and regional climate modelling, we show that drainage of surface meltwater to the bed of outlet glaciers on the Antarctic Peninsula occurs and triggers rapid ice flow accelerations (up to 100% greater than the annual mean). This provides a mechanism for this sector of the Antarctic Ice Sheet to respond rapidly to atmospheric warming. We infer that delivery of water to the bed tran- siently increases basal water pressure, enhancing basal motion, but efficient evacuation subsequently reduces water pressure causing ice deceleration. Currently, melt events are sporadic, so efficient subglacial drainage cannot be maintained, resulting in multiple short- lived (<6 day) ice flow perturbations. Future increases in meltwater could induce a shift to a glacier dynamic regime characterised by seasonal-scale hydrologically-driven ice flow variations. 1 Department of Geography, The University of Sheffield, Sheffield, UK. 2 School of Geography and Sustainable Development, University of St Andrews, Andrews, UK. 3 Institute for Marine and Atmospheric research Utrecht, Utrecht University, Utrecht, The Netherlands. *email: j.ely@sheffield.ac.uk NATURE COMMUNICATIONS | (2019) 10:4311 | https://doi.org/10.1038/s41467-019-12039-2 | www.nature.com/naturecommunications 1 ARTICLE NATURE COMMUNICATIONS | https://doi.org/10.1038/s41467-019-12039-2 he melting of snow and ice creates networks of meltwater 61°W Cayley Glacier – streams and ponds on glacier and ice-sheet surfaces1 3. a 62°S b T fl Surface meltwater is known to in uence the dynamics of many glaciers4–7 and portions of the Greenland Ice Sheet8,9.At these locations, drainage of surface water to the ice-bed interface Velocity impacts basal water pressure, leading to variations in ice motion 10 km – magnitude on sub-daily to decadal timescales1,4,7 13. This mechanism cou- (m a–1) 66°S fl Drygalski Glacier ples ice ow to atmospheric processes over a range of timescales >1500 c down to the sub-diurnal level, making affected ice masses respond 72°W 68°W 64°W rapidly to changes in atmospheric circulation patterns induced by climate change9. Surface meltwater has recently been reported to be widespread 0 across the Antarctic Ice Sheet3. Antarctic-wide melting is pro- 10 km jected to double by 2050, with the greatest increases in meltwater Hektoria Glacier production expected across the Antarctic Peninsula, where d atmospheric warming is already increasing surface melting14,15. Surface meltwater had catastrophic consequences for some ice shelves, where meltwater expedites ice-shelf disintegration by 62°W hydraulically driven fracture16. However, whether surface melt- water impacts the behaviour of grounded ice in Antarctica, implying a rapid and direct coupling of ice dynamics with 10 km atmospheric conditions, is currently unknown17. Jorum Glacier In this paper, we use high-resolution satellite-derived ice e velocity data, optical satellite imagery and regional climate modelling to study five Antarctic Peninsula outlet glaciers and ascertain whether surface meltwater drains to the bed of these 65°S 6 km glaciers, influencing dynamics at the ice-bed interface. The data reveal that multiple short-lived (<6 day) rapid and large accel- Crane Glacier erations (in some cases 100% greater than mean annual ice f velocity) termed speed-up events occur. That the relative mag- nitude of speed-up events increases away from the marine mar- gin, and that tidal fluctuations, iceberg calving events and sea-ice break-up are asynchronous with speed-ups, leads us to rule-out marine processes as a cause. Instead, the spatial pattern of speed- 0210 040 8 km ups, their concurrence with periods of modelled surface melting, km and observations of potential routes for surface meltwater to 62°W reach the bed from optical satellite imagery are consistent with a Fig. 1 Mean ice velocity map and location of studied glaciers. a Mean fi surface meltwater trigger. For the rst time, we show that surface velocity magnitude across the study area, between October 2016 and mid- meltwater affects the dynamics of grounded ice in Antarctica, April 2018. Inset shows the location on the peninsula, and the black line is proving a mechanism by which atmospheric and ice-dynamic the grounding line45. b–f Landsat 8 satellite images of glacier surfaces. The processes are coupled over short-timescales. black boxes outline the regions of interest. Note the visible water on the glacier surfaces Results − a 400 m a 1 (100% greater than the annual mean) speed-up Ice velocity. We used feature tracking of Sentinel-1 radar imagery fi occurring 9 km from the grounding line of Hektoria Glacier to generate velocity maps at 6-day intervals for ve marine- during the November 2017 event (Supplementary Fig. 4c). These terminating outlet glaciers on the Antarctic Peninsula (Fig. 1), data indicate that speed-up events were short-lived, typically during the period October 2016 to April 2018 (Methods). Four of lasting for 6 days or less. Most of the speed-up events were fol- the glaciers, Drygalski, Hektoria, Jorum and Crane, are on the lowed immediately by a slow-down before velocities return to eastern side of the Antarctic Peninsula, while Cayley Glacier is on below pre-event values. No significant slow-down events were the west side (Fig. 1). For each glacier, velocity was analysed detected in the absence of a speed-up. Slow-down and speed-up across a series of 1 km square regions of interest (ROIs), events were similar in duration (≤6 days), but the velocity change extending inland from the glacier’s grounding-line. Glacier- fl associated with the slow-downs was typically smaller (Fig. 2a, c; averaged ROI velocities reveal three types of velocity uctuation. Supplementary Figs. 1–4). Such speed-up events have not pre- First, large-scale seasonal variations, which decrease in magnitude viously been reported from Antarctica. away from the ocean. Second, background velocity fluctuations of ≈50 m a−1 magnitude. Finally, short-lived rapid accelerations in ice velocity to values >20% greater than the annual mean Marine processes. Two sets of processes could be the cause of (Fig. 2a). The latter of these we refer to here as speed-up events speed-up events, either marine processes and/or surface melt- and are the focus of this paper. Despite being separated by over water drainage to the base of the glacier. Marine processes, such 100 km, all five glaciers experienced near-synchronous speed-up as tidal fluctuations18, seasonal sea-ice break-up19 or iceberg events in March 2017, November 2017 and March 2018 (Fig. 2a); calving events20, can all lead to changes in ice buttressing forces. for example, velocities averaged across all ROIs on Drygalski If these processes were the trigger for the observed speed-up Glacier (Fig. 1c) increased by 300 m a−1 (≈23% greater than the events (Fig. 2), we would expect an increase in the relative annual mean) in November 2017 (Fig. 2a). Larger accelerations magnitude of speed-up events closer to the glacier terminus and were recorded in individual ROIs (Supplementary Figs. 1–4), with changes in marine conditions to coincide with speed-up events. 2 NATURE COMMUNICATIONS | (2019) 10:4311 | https://doi.org/10.1038/s41467-019-12039-2 | www.nature.com/naturecommunications NATURE COMMUNICATIONS | https://doi.org/10.1038/s41467-019-12039-2 ARTICLE a c 1800 2600 Drygalski 2 km inland Cayley 3 km inland 1600 Crane 2400 4 km inland Hektoria 5 km inland Jorum 1400 2200 1200 2000 –1) –1) 1000 1800 800 1600 Speed (m a Speed (m a 600 1400 400 1200 200 1000 0 800 Oct16 Jan17 Apr17 Jul17 Oct17 Jan18 Apr18 Oct16 Jan17 Apr17 Jul17 Oct17 Jan18 Apr18 b d 20 Definite melt 30 Possible melt ) ) No melt –1 –1 15 Cloud Darkness 20 10 Snowmelt Snowmelt 5 10 (mm w.e. day (mm w.e. (mm w.e. day (mm w.e. 0 0 Oct16 Jan17 Apr17 Jul17 Oct17 Jan18 Apr18 Oct16 Jan17 Apr17 Jul17 Oct17 Jan18 Apr18 Fig. 2 Velocity and modelled surface melt of studied glaciers. a Velocity variations at multiple glaciers display prominent and coincident speed-ups, defined in the text, indicated by dashed lines, which correspond with spikes in modelled melt b.
Details
-
File Typepdf
-
Upload Time-
-
Content LanguagesEnglish
-
Upload UserAnonymous/Not logged-in
-
File Pages9 Page
-
File Size-