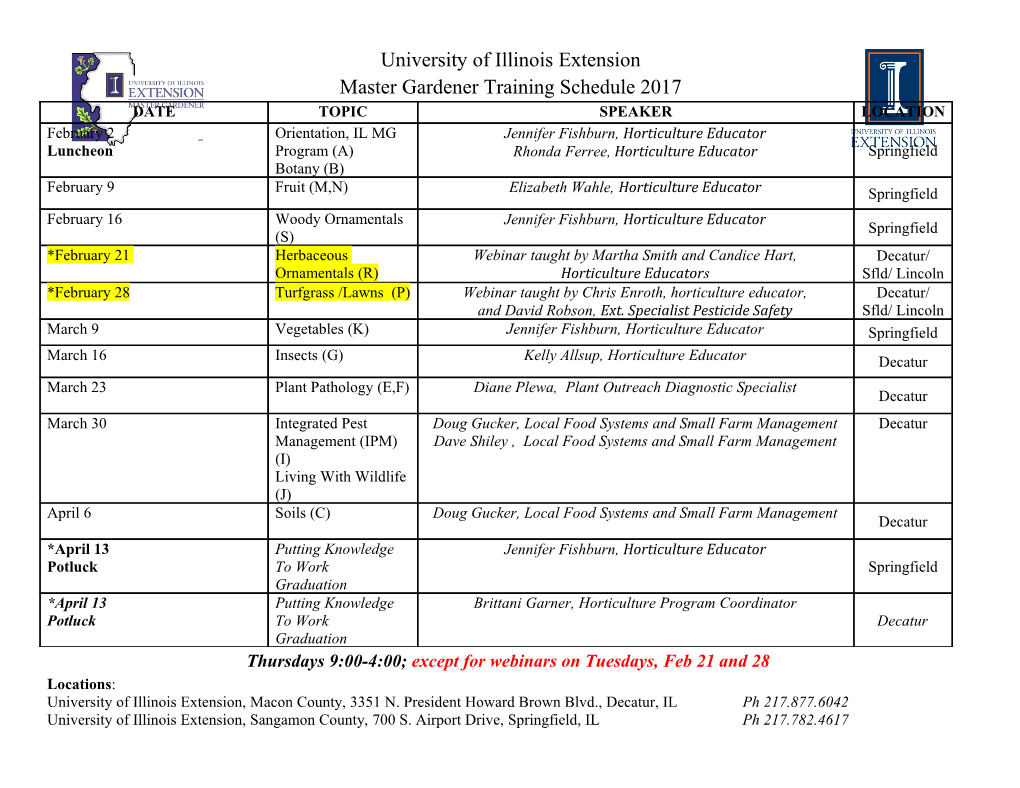
Geophysical Research Letters RESEARCH LETTER The role of South Pacific atmospheric variability 10.1002/2017GL073475 in the development of different Key Points: types of ENSO • South Pacific atmospheric variability, namely, the South Pacific Oscillation (SPO), plays a key role in the Yujia You1 and Jason C. Furtado1 prediction of ENSO flavors • The sources of variability of the SPO 1 are both intrinsic and also forced by School of Meteorology, University of Oklahoma, Norman, Oklahoma, USA anomalous convection in the central tropical Pacific • The SPO potentially plays a significant Abstract Recent advances in tropical Pacific climate variability have focused on understanding the role in climate variability on multiple development of El Niño–Southern Oscillation (ENSO) events, specifically the types or “flavors” of ENSO timescales: seasonal, interannual, and multidecadal (i.e., central versus eastern Pacific events). While precursors to ENSO events exist, distinguishing the particular flavor of the expected ENSO event remains unresolved. This study offers a new look at ENSO predictability using South Pacific atmospheric variability during austral winter as an indicator. The positive Supporting Information: • Supporting Information S1 phase of the leading mode of South Pacific sea level pressure variability, which we term the South Pacific Oscillation (SPO), exhibits a meridional dipole with with a(n) (anti)cyclonic anomaly dominating the subtropics (extratropics/high latitudes). Once energized, the cyclonic anomalies in the subtropical node Correspondence to: Y. You, of the SPO weaken the southeasterly trade winds and promote the charging of the eastern equatorial [email protected] Pacific Ocean, giving rise to eastern Pacific ENSO events. Indeed, the type of ENSO event can be determined accurately using only the magnitude and phase of the SPO during austral winter as a predictor (17 out of Citation: 23 cases). The SPO may also play a role in explaining the asymmetry of warm and cold events. Collectively, You, Y. and J. C. Furtado (2017), our findings present a new perspective on ENSO-South Pacific interactions that can advance overall The role of South Pacific atmospheric understanding of the ENSO system and enhance its predictability across multiple timescales. variability in the development of different types of ENSO, Geophys. Res. Lett., 44, doi:10.1002/2017GL073475. 1. Introduction Received 16 MAR 2017 Accepted 28 JUN 2017 The El Niño-Southern Oscillation (ENSO) is one of the most prominent year-to-year fluctuation in the Earth Accepted article online 5 JUL 2017 climate system and influences tropical convective processes along with the global atmospheric circulation. In recent decades, increasing interests have been placed on the diversity of ENSO events—i.e., eastern Pacific (EP) versus central Pacific (CP) El Niño events [Ashok et al., 2007; Yu et al., 2012]—due to their distinct remote impacts. For CP events, the sea surface temperature anomaly (SSTa) extends from Baja California southwest- ward to the central equatorial Pacific and thereafter remains and amplifies in situ [Kao and Yu, 2009; Kug et al., 2009; Yu et al., 2010; Capotondi et al., 2015]. The evolution of EP events features rapid warming of SSTs over the eastern tropical Pacific [e.g., Wyrtki, 1975; Rasmusson and Carpenter, 1982] explained by theories like the delayed oscillator [e.g., Suarez and Schopf, 1988] and the discharge-recharge mechanisms [e.g., Jin, 1997]. The two types of ENSO events may be distinguishable, although some studies suggest that ENSO flavors come in a nondiscrete continuum [e.g., Johnson, 2013; Karnauskas, 2013]. Aside from the mechanisms of formation, research on the precursors to specific ENSO events has also been conducted. Particularly, the presence of the North Pacific Oscillation (NPO), characterized by a large-scale meridional sea level pressure (SLP) anomaly (SLPa) dipole between roughly Alaska and Hawaii [e.g., Rogers, 1981; Linkin and Nigam, 2008], serves as an important precursor for ENSO ∼9 months before the event [Chang et al., 2007]. Dynamically, the anomalous circulations associated with the southern node of the NPO modulate the off-equatorial trade winds, charge the central equatorial heat content [Anderson et al., 2013a; Anderson and Perez, 2015], and may initiate an ENSO event [e.g., Vimont et al., 2003; Chiang and Vimont, 2004]. However, there is little evidence currently that the NPO precursor favors one type of ENSO over the other [e.g., Ding et al., 2015; DiLorenzoetal., 2015], although it may influence the longitude of the warmest SSTa [Anderson et al., 2013b]. Turning to the Southern Hemisphere, Zhang et al. [2014] argue for the impor- tance of the South Pacific Meridional Mode (SPMM) in initiating EP ENSO events, although the origin of the ©2017. American Geophysical Union. SPMM is unclear. Extratropical South Pacific ocean dynamics may also play an important role in extended All Rights Reserved. predictability of ENSO events, with off-equatorial wind stresses impacting tropical Pacific thermocline depths YOU AND FURTADO SOUTH PACIFIC OSCILLATION AND ENSO 1 Geophysical Research Letters 10.1002/2017GL073475 [McGregor et al., 2009a, 2009b]. Debate on cause and effect between South Pacific atmospheric variability and ENSO remains an open research question [e.g., Jin and Kirtman, 2009]. The present study examines the linkages between the extratropical South Pacific atmosphere and tropical Pacific SST variations associated with different types of ENSO. Our findings indicate that a prominent mode of South Pacific atmospheric variability is likely a favorable condition for the formation of EP versus CP ENSO events. Thus, this work can be viewed as a stepping stone to recognize the importance that the South Pacific plays in generation of ENSO events, mirroring (and adding to) existing results of the role of North Pacific atmospheric variability on tropical Pacific climate variability. 2. Data and Methods Monthly mean atmospheric fields (SLP, 10 m winds) from the National Centers for Environmental Prediction/ National Center for Atmospheric Research (NCEP/NCAR) reanalysis 1 [Kalnay et al., 1996] from 1948 to 2015 are employed in this study. SST and subsurface ocean data over the same period are taken from the Hadley Centre Global Sea Ice and Sea Surface Temperature data set [Rayner et al., 2003] and the Ocean Reanalysis System 4 European Centre for Medium-Range Weather Forecasts (ECMWF) ocean analysis [Balmaseda et al., 2013]. Anomalies of all fields are derived by subtracting the long-term climatology (1981–2010) for a particular month from the monthly mean values. All data are linearly detrended, and a 3 month running mean is applied before statistical analyses are conducted. Several climate indices are derived and used in this study. The cold tongue index (CTI; SSTa averaged over 6∘S–6∘N, 180∘W–90∘W), the Niño-3 (5∘S–5∘N, 150∘W–90∘W), and the Niño-4 (5∘S–5∘N, 160∘E–150∘W) indices are used to represent ENSO variability. An El Niño (A La Niña) is defined as a year in which the December–February (DJF) Niño-3 index or Niño-4 index exceeds (is lower than) 0.5∘C(−0.5∘C).AnEP(CP) ENSO event is then classified using the same criterion as Yehetal.[2009]: i.e., an ENSO event during which the DJF Niño-3 index is greater (less) than the DJF Niño-4 index. Linear regression, compositing, and empirical orthogonal function (EOF) analyses are the primary statistical tools used in this study. For some analyses, we are interested in removing the linear dependence of a time series y(t) with some other climate mode or variable x(t) to examine residual variability. Calling this residual, y−rx: y−rx(t)=y(t)−[ + × x(t)], where and are found through least squares fitting. Thus, y−rx(t) is, by definition, uncorrelated with x(t). Statistical significance of regression coefficients is determined by a two- tailed Student’s t test with an effective degrees of freedom N∗ computed in Bretherton et al. [1999] as follows: 1 − r r N∗ = N 1 2 (1) 1 + r1r2 where N is the total sample size and r1 and r2 are the lag 1 correlations of the index and field used in the particular analysis, respectively. Finally, in investigating oceanic pathways linking the South Pacific to the tropical Pacific, we compute the depth-integrated meridional velocity V (i.e., Sverdrup transport) as in Anderson and Perez [2015] [ ] 1 y V ≈ − x (2) x y where x and y are the eastward and northward components of the wind stress, respectively, is the latitu- dinal gradient of the Coriolis parameter, and =1025 kg/m3 is the density of sea water. The wind stresses are 2 . . 3 computed as = CD airU , where CD = 0 0013 is the drag coefficient, air = 1 2 kg/m is the density of air, and U is the 10 m wind speed. 3. Results 3.1. The Leading Mode of South Pacific Atmospheric Variability We begin by examining the dominant modes of South Pacific atmospheric variability via EOF analysis of monthly mean South Pacific SLPa field over the domain 10∘S–45∘S, 160∘W–70∘W. Figure 1a shows the regres- sion of monthly SLPa (line contours), SSTa (shading), and 10 m wind anomalies (vectors) onto the standardized leading principal component (PC1) time series of monthly mean South Pacific SLPa. The leading mode explains YOU AND FURTADO SOUTH PACIFIC OSCILLATION AND ENSO 2 Geophysical Research Letters 10.1002/2017GL073475 Figure 1. (a) Regression of SLPa (contour, hPa), SSTa (shading, ∘C), and 10 m wind anomalies (vector, m s−1) onto the standardized PC1 time series of monthly mean South Pacific SLPa (i.e., the SPO index). Contour interval 0.4 hPa (line contours) and 0.1∘C (shading). Reference wind vector 0.5 m s−1. Solid (dashed) line contours indicate positive (negative) values. Zero contour omitted.
Details
-
File Typepdf
-
Upload Time-
-
Content LanguagesEnglish
-
Upload UserAnonymous/Not logged-in
-
File Pages9 Page
-
File Size-