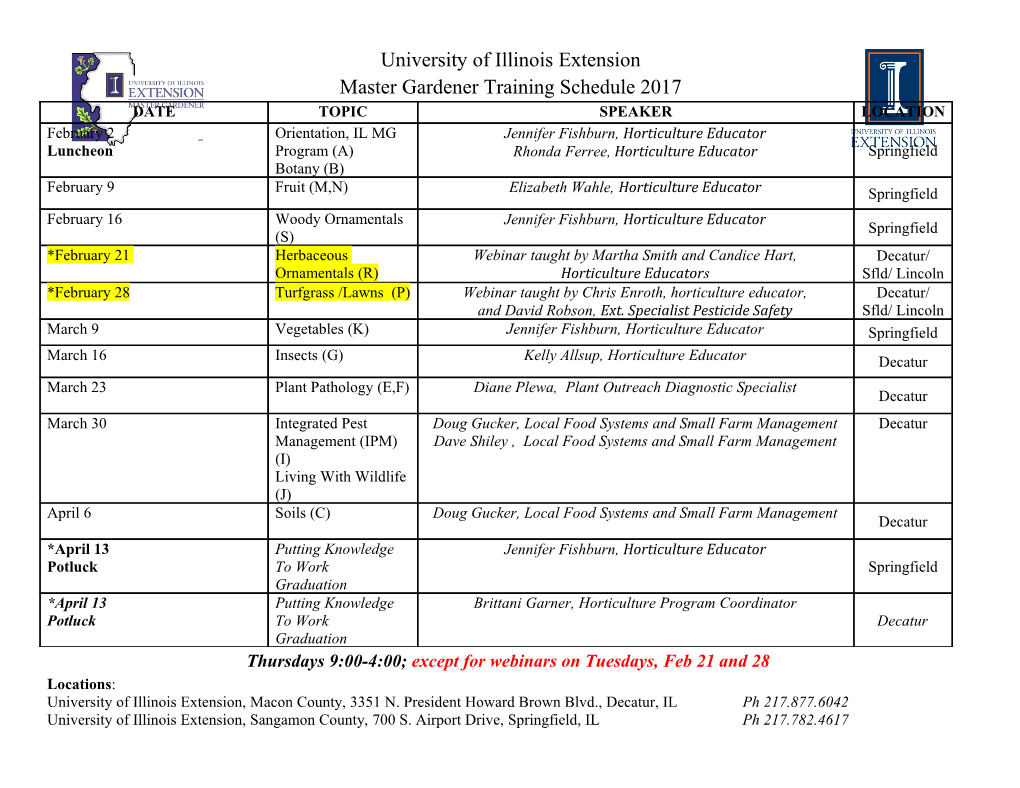
Expanding the Landscape of Biological Computation with Synthetic Multicellular Consortia Ricard V. Solé Javier Macia SFI WORKING PAPER: 2013-05-020 SFI Working Papers contain accounts of scientiic work of the author(s) and do not necessarily represent the views of the Santa Fe Institute. We accept papers intended for publication in peer-reviewed journals or proceedings volumes, but not papers that have already appeared in print. Except for papers by our external faculty, papers must be based on work done at SFI, inspired by an invited visit to or collaboration at SFI, or funded by an SFI grant. ©NOTICE: This working paper is included by permission of the contributing author(s) as a means to ensure timely distribution of the scholarly and technical work on a non-commercial basis. Copyright and all rights therein are maintained by the author(s). It is understood that all persons copying this information will adhere to the terms and constraints invoked by each author's copyright. These works may be reposted only with the explicit permission of the copyright holder. www.santafe.edu SANTA FE INSTITUTE Expanding the landscape of biological computation with synthetic multicellular consortia Ricard V. Sol´e∗1, 2, 3 and Javier Macia1, 2 1ICREA-Complex Systems Lab, Universitat Pompeu Fabra, Dr Aiguader 88, 08003 Barcelona, Spain 2Institut de Biologia Evolutiva, UPF-CSIC, Psg Barceloneta 37, 08003 Barcelona, Spain 3Santa Fe Institute, 1399 Hyde Park Road, Santa Fe NM 87501, USA Computation is an intrinsic attribute of biological entities. All of them gather and process infor- mation and respond in predictable ways to an uncertain external environment. Are these com- putations similar to those performed by artificial systems? Can a living computer be constructed following standard engineering practices? Despite the similarities between molecular networks as- sociated with information processing and the wiring diagrams used to represent electronic circuits, major differences arise. Such differences are especially relevant while engineering molecular cir- cuits in order to build novel functionalities. Among others, wiring molecular components within a cell becomes a great challenge as soon as the complexity of the circuit becomes larger than simple gates. An alternative approach has been recently introduced based on a non-standard approach to cellular computation. By breaking some standard assumptions of engineering design, it allows the synthesis of multicellular engineered circuits able to perform complex functions and open a novel form of computation. Here we review previous studies dealing with both natural and synthetic forms of computation. We compare different systems spanning many spatial and temporal scales and outline a possible space of biological forms of computation. We suggest that a novel approach to building synthetic devices using multicellular consortia allows us to expand this space in new directions. Keywords: Synthetic biology, cell computing, circuit design, evolution, robustness I. INTRODUCTION unreliable components within crowded cell interiors, self- renewing tissues, neuronal loss as well as uncertain en- vironments. A global view of how is this repertoire of Living systems are characterized by their potential solutions is related and how to define a space of possible for performing computations (Hopfield 1994; Bray 1995; forms of computation requires defining a proper theo- Arbib 1995; Amos 2004; Sol´eet al 2007; Nurse 2008; retical framework and a systematic comparison between Brenner 2012). Early evolution of life was marked by existing systems (Bray 1995). Understanding the simi- a plethora of events involving efficient energy use and larities as well as the differences between man-made com- an adequate exploitation of available matter to power puting artifacts and their natural counterparts has been their metabolic pathways and build and maintain their a central issue in the study of natural computing. We internal organization. But the real trick involved de- know from comparative biology that common solutions veloping mechanisms to gather, store, process and react are often found as a result of independent evolutionary to information in complex, adaptive ways. As it occurs paths (Conway Morris 2004). with computers, biological systems are information pro- cessors, and as complexity grows with evolution, more Information-processing systems provide a neat exam- complex computational tasks emerge. However, it has ple of the power of such convergent designs. Some of been well known since the beginnings of computation these systems, such as cortical networks processing visual that crucial differences exist in the way computations information, display an architecture that is no different are actually performed by living entities compared with from the solutions found by engineers designing paral- man-made artifacts. This was early identified as a ma- lel computers performing visual recognition tasks (Nel- jor issue when computers and brains were compared (von son and Bower 1990). Such convergence in evolved ver- Neumann 1956, 1958). The rise of molecular biology pro- sus planned designs is remarkable, since engineers were vided additional evidence for an unexpected robustness not getting any inspiration from cortical circuits. Simi- of cellular information processing suggesting that a whole lar scenarios have been found associated to wiring very range of biological systems exhibit such reliable compu- large scale integrated (VLSI) circuits under strong pack- tation capacity out from unreliable, noisy components ing constraints (Moses et al 2008) which are also followed (Kitano 2004, 2007). by brains (Bassett et al 2010; Sol´eet al., 2013). Biological systems have found through evolution dif- If such similarities were powerful enough, it could be ferent mechanisms to deal with molecular fluctuations, argued that a technology-inspired evolutionary theory of biological computation should be taken as a good starting point. If true, it would be possible to establish a mapping between man-made computational devices of increasing ∗corresponding author complexity and the repertoire of computationally mean- 2 a b c d e f FIG. 1 Electronic circuits (a) are the iconic representations of standard computing machines. In biology, computations are often distributed among individuals having a limited information about their neighbors but are able to generate stable large-scale structures which allow collective decisions to take place. In (b) we illustrate this with the choice performed by Physarum colonies, which are able to decide among different food sources (picture by Audrey Dussutour, see http://dussutou.free.fr/). Parallel computation is widespread in several important biological examples, where a fluid neural-like structure is involved, as it occurs with the immune system (c) where some particular cells, such as dendritic cells (c, from http://en.wikipedia.org/wiki/Dendritic cell) or ant colonies while solving computational problems, such as finding the shortest path among two alternatives (d, image courtesy of Guy Theraulaz). In systems where single-cell behavior is the dominant form of behavior, as it occurs for example with yeast cells (e) intracellular decision making networks exist. At the molecular scale (f) complex nanomachines, such as the DNA polymerase "read" DNA as a tape (picture generated using Pymol software). All these systems perform computations of some type, and their special characteristics make them inhabit different locations in the abstract landscape of biocomputation. 3 ingful living structures (see Sauro and Khodolenko 2004; based on a parallel exploration of a spatial domain un- Istrail et al 2007; Hogeweg 2002). A standard approach der a pattern-formation process (Adamatzky 2007; see to this is given by so called theoretical morphospaces, also Dussutour et al 2010). The role played by space an abstract way of geometrically characterize the pos- is particularly important when dealing with communi- sible spectrum of biological structures of a given class cation taking place among different individuals, wether (McGhee 2006). By defining a small number of axes (usu- cells or ants. Due to the constraints associated to spatial ally related to geometrical or physiological properties) it interactions, parallelism is a necessary condition for reli- is possible to see what part of the space is occupied, what able computation. Space provides a well-defined dimen- part is empty and look for an interpretation of such dis- sion for our morphospace. It allows multiple segregated tribution. The recent developments within the emergent components to interact locally with others, provides a field of synthetic biology (Bennett 1982; Tan et al 2007; natural source of modularization and it also defines a Benenson 2009) have created a new scenario where the deep connection between pattern formation and pattern manipulation of cellular systems provides a new oppor- recognition (Haken 2004, 1979). Back again to synthetic tunity of expanding the space of biological computations biology, engineering cell-cell communications in a spatial (Weiss et al 2003; Brenner 2012). Since such novel de- context allows to explore the formation of multicellular signs are not the result of evolutionary paths nor need systems (Kinkhabwala and Bastiaens 2010). to be restricted to standard forms of engineering, they Similarly, phenotypic diversity among cells involved in offer the potential for creating new classes of computa- a given process of pattern recognition,
Details
-
File Typepdf
-
Upload Time-
-
Content LanguagesEnglish
-
Upload UserAnonymous/Not logged-in
-
File Pages14 Page
-
File Size-