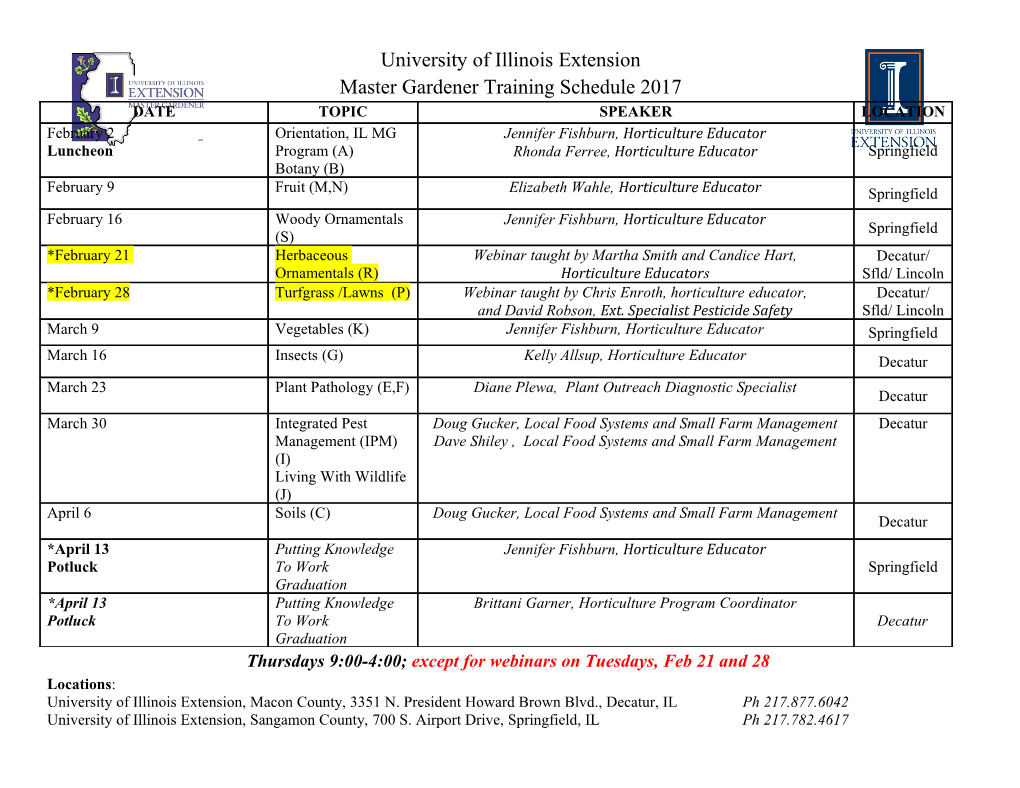
THE UNIVERSITY OF CHICAGO LOW LATITUDE ANDEAN GLACIERS: CLIMATE DRIVERS OF PAST, PRESENT, AND FUTURE CHANGES A DISSERTATION SUBMITTED TO THE FACULTY OF THE DIVISION OF THE PHYSICAL SCIENCES IN CANDIDACY FOR THE DEGREE OF DOCTOR OF PHILOSOPHY DEPARTMENT OF THE GEOPHYSICAL SCIENCES BY ANDREW GRADY OROS MALONE CHICAGO, ILLINOIS JUNE 2017 Copyright c 2017 by Andrew Grady Oros Malone All Rights Reserved TABLE OF CONTENTS LIST OF FIGURES . v LIST OF TABLES . vi ACKNOWLEDGMENTS . vii ABSTRACT . viii 1 INTRODUCTION . 1 1.1 Glacier-Climate Interactions . .4 1.2 Setting of Low Latitude Glaciers . .9 1.3 Dissertation Objectives . 14 2 CLIMATE DRIVERS OF TROPICAL ANDEAN GLACIER CHANGE . 17 2.1 Three Representative Tropical Glaciers . 21 2.2 Methods . 25 2.2.1 Regional-Scale Surface Energy and Mass Balance Modeling . 25 2.2.2 Model Inputs, Outputs, and Validation Methods . 30 2.2.3 Experiment 1: Mass Balance Response To Contemporary Climate Signal 33 2.2.4 Experiment 2: Mass Balance Response To Certain Climate Signals . 34 2.3 Results . 34 2.3.1 Model Output And Validation . 34 2.3.2 Modeled Year-To-Year Variability . 42 2.3.3 Modeled Year-To-Year Variability and Variability in the Climate Inputs 44 2.3.4 Modeled Year-To-Year Variability and Variability in the Specific Cli- mate Inputs . 47 2.3.5 Day-To-Day and Year-To-Year Melt Variability at Different Elevations and Associated Energy Fluxes and Climate Inputs . 53 2.4 Discussion . 56 2.4.1 Wet-Dry Contrast . 56 2.4.2 Drivers of Wet Tropical Glacier Change Across Temporal And Spatial Scales . 60 2.4.3 Tropical Glaciers and Mass Balance Asymmetries . 63 2.5 Concluding Remarks . 67 3 RECONSTRUCTIONS OF TROPICAL PALEOCLIMATES . 69 3.0.1 Quelccaya Ice Cap Setting and Glacial Chronology . 72 3.1 Methods . 74 3.1.1 Glacier Flow Model . 74 3.1.2 Surface Energy and Mass Balance Model . 75 3.1.3 Equilibrium Glacier Length Simulations (Experiments 1 & 3) . 76 3.1.4 Transient Glacier Length Simulations (Experiment 2) . 78 3.2 Results . 79 iii 3.2.1 Equilibrium Glacier Length Response . 79 3.2.2 Interannual Climate Variability Response . 81 3.2.3 Paleoclimate Reconstructions . 83 3.3 Discussion . 86 3.3.1 Climate Drivers of Glacier Length Change . 86 3.3.2 Little Ice Age (LIA) Tropical Climate Change . 89 3.3.3 Younger Dryas (YD) Tropical Climate Change . 91 3.4 Concluding Remarks . 93 4 HINDCASTS AND FORECASTS IN LIGHT OF MASS BALANCE NONLINEAR- ITIES . 95 4.1 Methods . 97 4.1.1 Transient Glacier Length Simulations . 98 4.1.2 Equilibrium Glacier Length Simulations . 100 4.2 Results . 101 4.2.1 ELA and Net Mass Balance Variability . 101 4.2.2 Length Fluctuations and Upslope Retreat . 104 4.3 Discussion . 106 4.3.1 Interannual Climate Variability and Mass Balance Nonlinearities . 106 4.3.2 The Universality of Mass Balance Nonlinearities . 109 4.3.3 Mass Balance Nonlinearities And Interpreting Glacier Length Changes 113 4.4 Concluding Remarks . 114 5 CONCLUSION . 116 A SURFACE ENERGY AND MASS BALANCE MODEL VARIABLES AND PARAM- ETERS . 121 B STATISTICS BETWEEN GLACIER-CLIMATE INTERACTION METRICS AND CLIMATE INPUTS . 123 REFERENCES . 126 iv LIST OF FIGURES 1.1 Location of world glaciers . .2 1.2 Surface energy fluxes on a glacier . .5 1.3 Accumulation and ablation season in different climate regimes . .9 1.4 Geographic data on tropical Andean glaciers . 12 1.5 Climate setting tropical Andean glaciers . 14 2.1 Three case study tropical glaciers . 23 2.2 Simulated vertical mass balance profiles . 37 2.3 Volc´anAntisana measured and modeled net mass balance comparison . 40 2.4 Simulated net mass balance variability . 43 2.5 Simulated contemporary ELA variability . 44 2.6 Net mass balance variability and temperature and precipitation variability . 45 2.7 ELA variability and temperature and precipitation variability . 47 2.8 Net mass balance variability for different climate inputs . 49 2.9 ELA variability for different climate inputs . 52 2.10 Melt fraction versus accumulation rate . 58 2.11 Mass balance at the mean ELA . 65 3.1 Relationship between tropical SST and free atmosphere temperature changes . 71 3.2 Glacial geology of the Quelccaya Ice Cap, Peru . 73 3.3 Sensitivity of the Huancan´eglacier length to climate changes . 80 3.4 Quelccaya Ice Cap response to climate variability . 82 3.5 Possible paleoclimates for Little Ice Age and Younger Dryas moraines at the QIC 84 3.6 Little Ice Age tropical paleoclimate reconstructions . 90 3.7 Younger Dryas tropical paleoclimate reconstruction . 92 4.1 Simplified glacier response to climate variability . 100 4.2 ELA and mass balance response to climate variability . 103 4.3 Transient and mean glacier length response to climate variability . 105 4.4 Cartoon of mass balance nonlinearity due to temperature variability . 108 4.5 Mass balance anomalies driven by climate variability . 110 v LIST OF TABLES 2.1 Mass balance and ELA sensitivity parameters . 53 2.2 Daily correlation between melt and climate variables or energy fluxes at wet tropical glaciers . 54 2.3 Interannual correlation between melt and climate variables at wet tropical glaciers 55 4.1 Representative mass balance gradients for tropical and mid latitude glaciers . 98 4.2 Percentage of times mass balance anomalies appear normally distributed . 112 A.1 Ch. 2 model variables . 121 A.2 Ch. 2 model parameters: radiative fluxes . 121 A.3 Ch. 2 model parameters: turbulent & other fluxes . 122 A.4 Ch. 2 model physical constants . 122 B.1 Ch. 2 Mass Balance & Climate Statistics . 124 B.2 Ch. 2 ELA & Climate Statistics . 125 vi ACKNOWLEDGMENTS I would like to thank my committee members for their support, insight, and assistance in this dissertation: Douglas MacAyeal (the University of Chicago), Ray Pierrehumbert (formerly the University of Chicago; now Oxford University), Dorian Abbot (the University of Chicago), and Edwin Kite (the University of Chicago). Ray, my former primary advisor, thank you for introducing me to tropical glaciers as a way to think about past and present climate change. Doug, my primary advisor, thank you for the support these last three years, challenging me to think deeper and helping me to move from big and interesting problems to tangible solutions. I would also like to acknowledge the wonderful faculty, graduate students, and staff in the Department of the Geophysical Sciences. Special thanks to Daniel Koll, a great friend and keen editor of manuscripts and poster drafts, and to Lei Wang, an awesome officemate with profound knowledge about all things dealing with fluid dynamics and climate. Also to Miquela, Bethany, and Lily, for helping me keep my focus and sanity, in part though the marvels of dark chocolate. Beyond the University of Chicago community, I am deeply grateful for the support of the greater geological and climatological communities. Thomas Lowell (University of Cincin- nati), thank you for helping me think deeply about the glacial world and climate change and for actively supporting collaborations between the glacial modeling and geology communities. Also, Meredith Kelly (Dartmouth College) and Yarrow Axford (Northwestern University), thank you for encouragement and insight into how we know what we know about past cli- mates; I look forward to future collaborations! Also to the support from the National Science Foundation in funding a portion of this research through EAR-1003686. Finally, my utmost gratitude to my wife, Rachel Daley, for being so supportive and loving as I worked for many months on this dissertation. And many thanks to my parents for their unwavering encouragement and support. vii ABSTRACT Tropical Andean glaciers are an essential component of the alpine Earth system providing vital water resources for life in their vicinity. Glaciers are also archives of past climate change and barometers of present climate change, and tropical glaciers can provide a much- needed additional low-latitude terrestrial paleoclimate proxy. Despite their societal and scientific importance, the study of tropical glaciers is nascent. This dissertation provides new perspectives on the climate components most important for tropical glacier change, illustrates that records of past tropical glacier changes can be used as a quantitive paleoclimate proxy, and provides a framework for a more rigorous interpretation of tropical glacier advances and retreats as a climate proxy. A regional-scale surface energy and mass balance model is developed and implemented to quantify tropical glacier response to different types of climate change. The mass balance evolution from thermal year (July - June) 1980 - 2009 CE is simulated, and the dominant input climate variables are determined. The dominant climate drivers of glacier change at tropical Andean glaciers vary, depending on the regional climate setting and in particular the amount of annual precipitation. For both wet inner tropical glaciers and wet outer trop- ical glaciers, interannual temperature variability is the dominant climate forcing mechanism for equilibrium line altitude (ELA) or mass balance variability. For dry tropical glaciers, precipitation variability is the dominant variable for mass balance and ELA variability. For tropical glaciers, all-wave radiation (i.e. net shortwave and longwave radiation) is the dom- inant source of available melt energy, and at wet tropical glaciers, which is the classification type of the majority of Andean glaciers, temperature is able to modulate the all-wave ra- diation from year-to-year. By dictating the phase of precipitation, temperature determines the surface albedo and absorbed shortwave radiation at the lowest extents of the glacier, and this relationship is so strong that it can define the glacier-wide mass balance. Thus, for wet tropical glaciers, observations of mass balance or length changes primarily reflect temperature change. viii A coupled ice flow and surface mass balance model is implemented at the world's largest tropical ice mass, the Quelccaya Ice Cap, Peru, to invert the Little Ice Age and Younger Dryas aged moraines into quantitive paleoclimate proxies.
Details
-
File Typepdf
-
Upload Time-
-
Content LanguagesEnglish
-
Upload UserAnonymous/Not logged-in
-
File Pages148 Page
-
File Size-