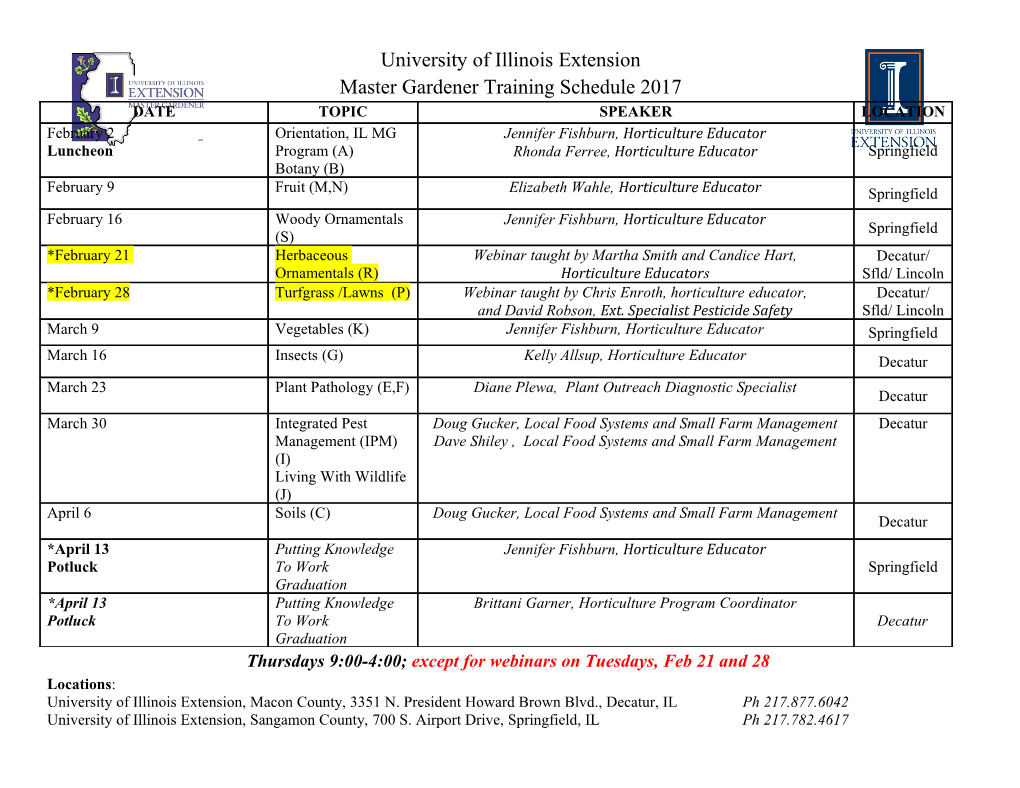
Molecular Electronics 1018 atoms of boron or arsenic per Improvements in our understanding of how molecules cubic centimeter, similar 5-nm diame- transport charge, and how they interface to the macro- ter, micron-long segments of silicon scopic world, are fueling new devices and applications. wires would have 15–20 dopant atoms, and a junction of two crossed wires would contain, on average, James R. Heath and Mark A. Ratner approximately 0.1 dopant atom. Con- sequently, field-effect transistors fab- magine molecular gears that oscillate against each other ricated at these wiring densities might exhibit non- Ias they are stimulated by the addition or removal of a sin- statistical, and perhaps unpredictable, behavior. Other gle electron,1 or a dominolike arrangement of molecules in concerns, such as the gate oxide thickness, power con- which a small perturbation on one end initiates a molecu- sumption (just from leakage currents through the gate lar-mechanical cascade that ripples through the whole as- oxide), and fabrication costs, also highlight the difficulty sembly in a predetermined fashion.2 Imagine a wire in of scaling standard electronics materials to molecular di- which the presence or absence of a single atom dominates mensions.6 At device areas of a few tens of square nanome- the electrical conductivity,3 or in which a biomolecular ters, molecules have an inherent attractiveness because of recognition process is used to open up new conductivity their size, because they represent the ultimate in terms of pathways.4 These descriptions are respectively those of a atomic control over physical properties, and because of the molecular-mechanical switch that forms the basis of a ran- diversity of properties—such as switching, dynamic or- dom access memory circuit, a molecular-based three-input ganization, and recognition—that can be achieved through mechanical Boolean sorter, a single-molecule electrically or such control. magnetically gated switch, and a single-stranded DNA wire Although molecular electronics has been the subject that is “turned on” by hybridization of the DNA into the of research for some time, over the past few years a num- double-stranded form. These molecular systems, and a host ber of synthetic and quantum chemists, physicists, engi- of other equally diverse chemical species, are principal ac- neers, and other researchers have sharply increased the tors in the rapidly emerging field of molecular electronics. ranks of this field. Several new molecular-electronic sys- Molecules have not historically played a prominent tems, analytical tools, and device architectures have been role in electronic devices. Ten years ago, chemical applica- introduced and explored. As a result, the basic science on tions were limited to the use of small molecules such as which a molecular electronics technology would be built is silanes (SixHy) and germanes (GexHy) as thin-film precur- now unfolding, and the science and applications that are sors or as the components of etching processes, resist pre- emerging are tremendously exciting. For example, current cursors, packaging materials, and the like. Engineered in- research is using molecules in such electronics applica- organic insulators, semiconductors, and metals were the tions as interconnects, switches, rectifiers, transistors, heart of the industry, and the fundamental knowledge that nonlinear components, dielectrics, photovoltaics, and gave birth to the integrated circuit was appropriately cred- memories. Excellent reviews of this field are available.7 In ited back to the fundamental solid-state physics that was this article, we focus on the current state of the art in one largely developed in the mid-20th century. area of molecular electronics, with an emphasis on the re- Over the past decade, the picture has not changed lationship between molecular structure and electrical con- much. Conducting polymers have emerged as a real, albeit ductance and on the use of molecules for computational ap- still minor, technology. However, over the next 10–20 years, plications. The essential science in these areas reflects the molecules may be increasingly viewed not just as the start- broader field of molecular electronics, and although cer- ing points for bulk electronic materials, but as the active tain fundamental challenges have been faced, many oth- device components within electronic circuitry (see, for in- ers must still be overcome. stance, figure 1). Although this possibility is hardly a fore- gone conclusion, a number of fundamental issues favor the Why molecular electronics? development of a true molecular-based electronics. Essentially all electronic processes in nature, from photo- Consider the lattice of nanowires5 in the electron mi- synthesis to signal transduction, occur in molecular struc- crograph of figure 2. Each wire is 5 nm in diameter, and tures. For electronics applications, molecular structures the lattice constant is 15 nm. At a typical doping level of have four major advantages: ̈ Size. The size scale of molecules is between 1 and Jim Heath is the Elizabeth W. Gilloon Professor and Professor of 100 nm, a scale that permits functional nanostructures Chemistry at the California Institute of Technology in Pasadena, with accompanying advantages in cost, efficiency, and California. Mark Ratner is the Charles E. and Emma H. Morrison power dissipation. Professor of Chemistry at Northwestern University in Evanston, ̈ Assembly and recognition. One can exploit specific Illinois. intermolecular interactions to form structures by nano- scale self-assembly. Molecular recognition can be used to © 2003 American Institute of Physics, S-0031-9228-0305-020-8 May 2003 Physics Today 43 a b 2.0 1 mm 1.0 mm 0 Figure 1. Molecular electronics devices. (a) This optical micrograph shows a collection of 64-junction molecular circuits, fabricated by a combination of soft-imprinting techniques for the wires and chemical assembly for the molecules at the wire intersections. (b) An atomic force micrograph of one of the circuits, which could be used either as a random access memory or as a combination logic and memory circuit. The molecules used in this circuit are bistable [2]rotaxanes. (After Y. Chen et al., ref. 13. Courtesy of Stan Williams, Hewlett–Packard Co.) modify electronic behavior, providing both switching and wrong for molecules because of the localized nature of most sensing capabilities on the single-molecule scale. molecular electronic states. ̈ Dynamical stereochemistry. Many molecules have mul- Consider the energy diagrams of figure 3, in which tiple distinct stable geometric structures or isomers (an ex- four types of molecular-electronic junctions are repre- ample is the rotaxane molecule in figure 3d, in which a rec- sented, with examples of molecular structures. In figure tangular slider has two stable binding sites along a linear 3a, one electrode functions as an electron donor and the track). Such geometric isomers can have distinct optical other as an electron acceptor. The electrodes are bridged and electronic properties. For example, the retinal mole- by a linear chain (an alkane). In 1961, Harden McConnell cule switches between two stable structures, a process that wrote down the rate constant for electron transfer across ⊂ –bl transduces light into a chemoelectrical pulse and allows a molecular bridge: kET Ae , where l is the bridge length vision. and b is an energy-dependent parameter characterizing ̈ Synthetic tailorability. By choice of composition and the molecule. For alkanes up to a certain length and for geometry, one can extensively vary a molecule’s transport, small applied voltages, this approximation works well: binding, optical, and structural properties. The tools of mo- Current through a junction decreases exponentially with lecular synthesis are highly developed. increasing chain length, and the alkane effectively serves Molecules have disadvantages, though, such as insta- as a simple energy barrier separating the two electrodes. bility at high temperatures. But overall, the four advan- The possible mechanisms for electron transport are tages render molecules ideal for electronics applications, much richer for the electron donor-bridge-electron accep- as Richard Feynman noted in his famous 1959 speech, tor (DBA) molecular junction of figure 3b. DBA complexes “There is Plenty of Room at the Bottom.” serve as models for understanding how charge transport mechanisms in solution translate into the conductivity of Mechanisms of molecular charge transport solid-state molecular junctions. In DBA complexes, the The synthesis and characterization of molecules are en- donor and acceptor sites are part of the molecule, and the deavors typically conducted in solution. Decades of re- lowest unoccupied sites on the donor and acceptor compo- search have given chemists intuitive models that serve as nents are separated from one another by a bridging com- a guide for using synthetic control to design physical prop- ponent that has molecular orbitals of differing energy. In erties into a molecule. A key challenge in molecular elec- a process called electron-type superexchange, electrons tronics is to translate that solution-phase intuition into a that tunnel from the right electrode into the acceptor state solid-state device setting. Certain molecular structures when a bias is applied may coherently transfer to the donor have emerged as models for relating intramolecular elec- state before tunneling to the left electrode. Alternatively, tron transfer
Details
-
File Typepdf
-
Upload Time-
-
Content LanguagesEnglish
-
Upload UserAnonymous/Not logged-in
-
File Pages7 Page
-
File Size-