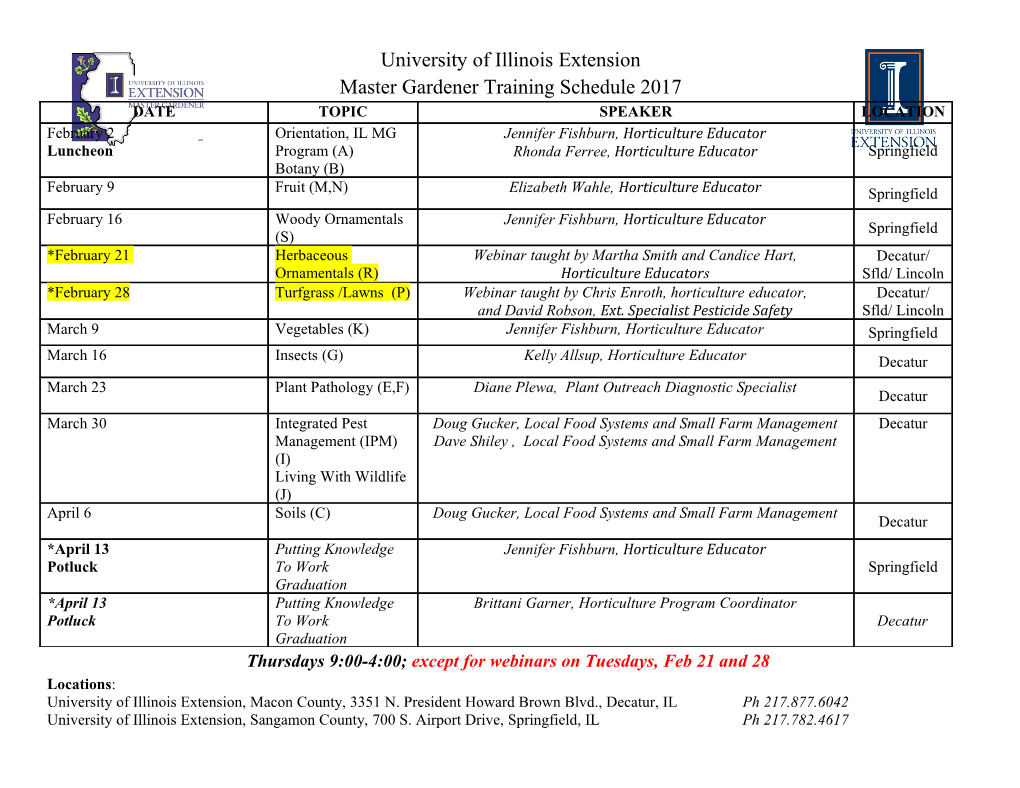
Proc. Natl. Acad. Sci. USA Vol. 96, pp. 85–90, January 1999 Biochemistry Characterization of functionally active subribosomal particles from Thermus aquaticus (ribosomeyrRNAyribosomal proteinsypeptidyl transferase) PHILIPP KHAITOVICH*, ALEXANDER S. MANKIN*†,RACHEL GREEN‡,LAURA LANCASTER‡, AND HARRY F. NOLLER‡ *Center for Pharmaceutical Biotechnology, myc 870, University of Illinois, 900 South Ashland Avenue, Chicago, IL 60607; and ‡Center for Molecular Biology of RNA, Sinsheimer Laboratories, University of California, Santa Cruz, CA 95064 Contributed by Harry F. Noller, November 2, 1998 ABSTRACT Peptidyl transferase activity of Thermus been shown to catalyze the peptidyl transferase reaction (see aquaticus ribosomes is resistant to the removal of a significant ref. 8 for review). number of ribosomal proteins by protease digestion, SDS, and Evolutionary arguments (reviewed in ref. 9), the discovery phenol extraction. To define the upper limit for the number of of ribozymes (10, 11), and a growing appreciation that rRNA macromolecular components required for peptidyl trans- plays an important functional role in translation have focused ferase, particles obtained by extraction of T. aquaticus large attention on 23S rRNA as a prime candidate for this catalytic ribosomal subunits were isolated and their RNA and protein role (12, 13). In vitro reconstitution has demonstrated that 23S composition was characterized. Active subribosomal particles rRNA is essential for the peptidyl transferase activity of 50S contained both 23S and 5S rRNA associated with notable subunits (6). Subsequent tRNA affinity labeling and footprint- amounts of eight ribosomal proteins. N-terminal sequencing ing experiments together with studies of antibiotic-resistance of the proteins identified them as L2, L3, L13, L15, L17, L18, mutations implicated domain V of 23S rRNA as an element of L21, and L22. Ribosomal protein L4, which previously was the peptidyl transferase center (8, 14). Nevertheless, most thought to be essential for the reconstitution of particles active attempts to demonstrate catalytic activity for isolated rRNA in peptide bond formation, was not found. These findings, were unsuccessful (see, however, refs. 15 and 16). One possible together with the results of previous reconstitution experi- explanation for this failure is that the active conformation of ments, reduce the number of possible essential macromolec- the RNA might be lost during protein extraction. Accordingly, ular components of the peptidyl transferase center to 23S rRNA from thermophilic organisms, which would be expected rRNA and ribosomal proteins L2 and L3. Complete removal to have a more robust structure, might have a better chance to of ribosomal proteins from T. aquaticus rRNA resulted in loss maintain its functional conformation upon removal of ribo- of tertiary folding of the particles and inactivation of peptidyl somal proteins. Indeed, peptidyl transferase activity of ribo- transferase. The accessibility of proteins in active subriboso- somes from the thermophilic bacteria Thermus aquaticus is mal particles to proteinase hydrolysis was increased signifi- resistant to vigorous protein-extraction procedures, including cantly after RNase treatment. These results and the observa- treatment with proteinase K in the presence of SDS followed tion that 50S ribosomal subunits exhibited much higher by phenol extraction, but is highly sensitive to treatment with resistance to SDS extraction than 30S subunits are compatible ribonuclease (17). To define the upper limit for the number of with a proposed structural organization of the 50S subunit macromolecular components that are required for peptidyl involving an RNA ‘‘cage’’ surrounding a core of a subset of transferase activity, we analyzed the properties and composi- ribosomal proteins. tion of active particles obtained after treatment of T. aquaticus 50S ribosomal subunits with proteinase K, SDS, and phenol Peptidyl transferase, the enzymatic activity responsible for (KSP particles, for proteinase K, SDS, and phenol). We found catalysis of peptide bond formation, was shown to be an that the extracted particles contained 23S rRNA and 5S rRNA integral part of the large ribosomal subunit more than 30 years associated with eight ribosomal proteins and sedimented at ago (1). However, despite decades of research, it is still not 50S, indicative of a compactly folded structure. Conditions known which molecular components of the large subunit, leading to removal of the remaining proteins caused dissoci- containing 23S and 5S rRNAs associated with more than 30 ation of 5S rRNA and a shift to a slower sedimentation value ribosomal proteins, contribute directly to the catalytic reac- along with complete loss of peptidyl transferase activity. tion. Genetic studies have shown that mutants lacking indi- vidual large ribosomal subunit proteins are viable, indicating MATERIALS AND METHODS that at least 10 different ribosomal proteins are dispensable for peptidyl transferase activity (2). Extraction of ribosomal pro- Cell Growth and Ribosome Isolation. T. aquaticus was teins with high concentrations of salt showed that a significant grown in a modified Castenholz TYE medium (18) containing fraction of ribosomal protein could be removed without drastic 13 Castenholz salts, 13 Nitsch’s trace elements, 4 gyliter yeast effect on peptidyl transferase (3–5). Finally, reconstitution of extract, 8 gyliter bactopeptone, and 2 gyliter NaCl (17). Cells 5 large ribosomal subunits from individual components with were grown at 70°C with shaking to A600 0.6, harvested, and omission of one or more proteins demonstrated that no more stored at 270°C. For preparation of 35S-labeled ribosomes, than a limited number of proteins, L2, L3, and L4, can possibly cells were grown in medium containing 13 Castenholz salts, be involved in the catalysis of peptide bond formation (6, 7). 103 Nitsch’s trace elements, 0.2 gyliter bactopeptone, and 2 However, no isolated protein, or mixture of proteins, has ever mCiyml of a mixture of [35S]methionine and cysteine (specific activity, 1,000 Ciymmol; American Radiolabeled Chemicals, The publication costs of this article were defrayed in part by page charge St. Louis). Ribosomes and ribosomal subunits were prepared payment. This article must therefore be hereby marked ‘‘advertisement’’ in as described in ref. 17. accordance with 18 U.S.C. §1734 solely to indicate this fact. © 1999 by The National Academy of Sciences 0027-8424y99y9685-6$2.00y0 †To whom reprint requests should be addressed. e-mail: shura@ PNAS is available online at www.pnas.org. uic.edu. 85 86 Biochemistry: Khaitovich et al. Proc. Natl. Acad. Sci. USA 96 (1999) Preparation of KSP Particles. KSP particles were prepared SDS loading buffer (25 mM TriszHCl, pH 6.5y6 M ureay1% by treatment of 50S subunits with proteinase K in the presence 2-mercaptoethanoly10% glyceroly1% SDSy0.005% bromo- of SDS, followed by phenol extraction essentially as described phenol blue), heated for 2 min at 90°C, and analyzed by (17). Briefly, 5 A260 of T. aquaticus 50S subunits were resus- electrophoresis in 15% polyacrylamideySDSyurea gel (25). pended in 500 ml of buffer A (25 mM TriszHCl, pH 7.5y150 The gel was stained with Coomassie blue. y y mM NH4Cl 5 mM MgCl2) containing 1 mg ml proteinase K RNase and Protease Treatment of KSP Particles. Two A260 and 0.5% SDS and incubated for 1 hr at 37°C. An equal volume units of the particles were incubated for 15 min at 37°C in 30 of neutralized phenol was added, vortexed for 45 min at 4°C, ml of buffer A with or without RNase A (final concentration, and centrifuged in a microcentrifuge at 4°C for 3 min. The 10 mgyml). This was followed by addition of SDS and protein- phenol phase was removed and the aqueous phase was ex- ase K to final concentrations of 0.5% and 2 mgyml, respec- tracted four times with chloroform. KSP particles were pre- tively, and incubation for 30 min at 37°C. Untreated control cipitated with three volumes of ethanol for 1 hr at 275°C, KSP particles were incubated for 45 min at 37°C in buffer A. pelleted in a microcentrifuge for 10 min at 4°C, and resus- At the end of the incubation, two-thirds of the reaction mixture pended in 50 ml of buffer A. was mixed with 10 ml of SDS loading buffer and proteins were Particles were purified by sedimentation in a sucrose gra- analyzed by gel electrophoresis in 15% polyacrylamideySDS dient (10–40%) in buffer containing 25 mM TriszHCl, pH gel (23). The gel was stained with Coomassie blue. y y y 7.5 250 mM NH4Cl 10 mM MgCl2 6 mM 2-mercaptoethanol Sequencing of 5S rRNA. To confirm the identity of the in an SW 41 rotor (Beckman) for 15 hr at 26,000 rpm at 4°C. fast-migrating RNA band on the polyacrylamide gel, it was Gradients were fractionated through an ISCO flow spectro- eluted from the gel and sequenced by the reverse transcrip- photometer. Peak fractions were combined, precipitated with taseydideoxy method using the primer d(CCCGCACCGAC- three volumes of ethanol, resuspended in 50 ml of buffer A, and CTACTCTCC), which is complementary to the 39 end of T. 2 stored at 75°C. aquaticus 5S rRNA (26). Peptidyl Transferase Assay. Peptidyl transferase activity was assayed by using a peptidyl transferase reaction (19) with a full-length formyl-[3H]Met-tRNA as a donor substrate (20). In RESULTS a typical assay, 7.4 pmol (0.2 A260) of 50S subunits or KSP 50S subunits of T. aquaticus ribosomes sediment as a single particles was incubated with 2.5 pmol (25,000 cpm) formyl- peak during sucrose gradient centrifugation (Fig. 1A). Treat- 3 m z [ H]Met-tRNA in 40 l buffer containing 20 mM Tris HCl, pH ment of the subunits with proteinase K in the presence of SDS y y y 8.0 400 mM KCl 20 mM MgCl2 1 mM puromycin. Reactions followed by phenol extraction converted them into KSP par- m were initiated by addition of 20 l cold methanol and incu- ticles, which formed four peaks on a sucrose gradient.
Details
-
File Typepdf
-
Upload Time-
-
Content LanguagesEnglish
-
Upload UserAnonymous/Not logged-in
-
File Pages6 Page
-
File Size-