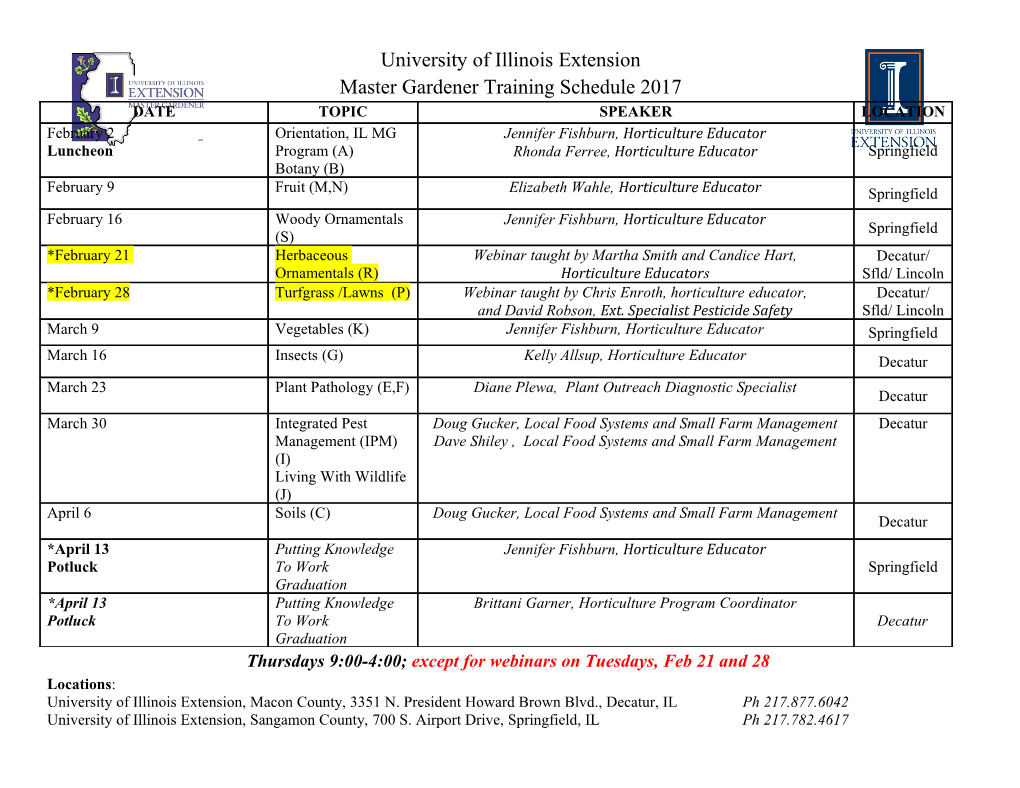
University of Southampton Research Repository ePrints Soton Copyright © and Moral Rights for this thesis are retained by the author and/or other copyright owners. A copy can be downloaded for personal non-commercial research or study, without prior permission or charge. This thesis cannot be reproduced or quoted extensively from without first obtaining permission in writing from the copyright holder/s. The content must not be changed in any way or sold commercially in any format or medium without the formal permission of the copyright holders. When referring to this work, full bibliographic details including the author, title, awarding institution and date of the thesis must be given e.g. AUTHOR (year of submission) "Full thesis title", University of Southampton, name of the University School or Department, PhD Thesis, pagination http://eprints.soton.ac.uk UNIVERSITY OF SOUTHAMPTON FACULTY OF MEDICINE School of Medicine Department of Cancer Sciences Manipulation of immunotherapy by targeting the inhibitory Fcγ receptor. by Emily Louisa Williams, BSc. (Hons) Thesis for the degree of Doctor of Philosophy June 2011 2 UNIVERSITY OF SOUTHAMPTON ABSTRACT FACULTY OF MEDICINE, SCHOOL OF MEDICINE, DEPARTMENT OF CANCER SCIENCES Doctor of Philosophy Manipulation of immunotherapy by targeting the inhibitory Fcγ receptor. Emily Louisa Williams BSc. (Hons) The inhibitory Fc receptor for IgG, FcγRII (CD32), has been shown to modulate in vivo cytotoxicity against tumour targets. Usually expressed on monocytes, macrophages and B lymphocytes, CD32 deficiency enhances the efficacy of some therapeutic mAb. Unfortunately, suitable reagents capable of specifically and exclusively binding mouse CD32 have been lacking, leading to an ignorance of how CD32 might be manipulated for therapeutic benefit in vivo. We generated a panel of mAb which are highly specific for CD32 that do not cross-react with any other Fc receptors, allowing us to study the potential of CD32 as a therapeutic target able to alter effector cell function and concurrent immunotherapy. In vitro analysis on murine lymphoma cell lines showed that the anti-CD32 mAb fall into two distinct categories; those which cause tyrosine phosphorylation and subsequent receptor activation (agonistic) and those which block receptor phosphorylation (antagonistic). Consequentially, these anti-CD32 mAb exert differing functions in a range of cellular and biochemical assays. Agonistic mAb trigger programmed cell death whilst antagonistic anti-CD32 mAb have relatively little effect. Interestingly, the agonistic mAb are able to block the magnitude and duration of the calcium signalling triggered by BCR engagement whilst antagonistic mAb potentiate these responses. Furthermore, these mAb were shown to trigger phagocytosis of antibody coated B-cells by murine bone marrow derived macrophages (BMDMs) and to enhance the phagocytic potential of BMDMs in vitro. The ability of these mAb to elicit therapeutic responses in a variety of different mouse models was however limited. Further study revealed that in wild-type mice the anti-CD32 mAb are rapidly consumed in comparison with other, similar, mAb. Together with our observation that the anti-CD32 mAb are internalised in vitro and in vivo, these data provide the rationale for the inadequate immunotherapy observed in our model systems. i ii Table of contents Abstract i Table of contents iii List of figures ix Lists of tables xii Declaration of Authourship xiii Dedication xv Acknowledgements xvii Abbreviations xix CHAPTER 1 Introduction 1 1.1. Introduction. 1 1.2. Immunotherapy. 2 1.2.1. Active immunotherapeutic strategies. 3 1.2.1.1. Cancer vaccination. 3 1.2.1.2. Immunostimulatory mAb. 5 1.2.2. Passive immunotherapeutic strategies. 6 1.3. Direct targeting mAb immunotherapy. 7 1.3.1. Antibody structure. 7 1.3.2. Development of mAb technology. 9 1.3.3. Effector mechanism of therapeutic mAb. 11 1.3.3.1. Induction of ADCC. 12 1.3.3.2. Induction of CDC. 12 1.3.3.3. Signal perturbation (PCD and altered endogenous signalling). 13 1.3.3.4. Additional anti-tumour functions. 15 1.3.4. Improving therapeutic mAb efficacy. 17 1.3.4.1. mAb conjugation. 17 1.3.4.2. Maximising FcγR:Fc interactions. 18 1.4. The Fc receptor family. 19 1.5. The Fcγ Receptors. 19 1.5.1. FcγR binding affinity for IgG. 21 1.5.2. The activatory-to-inhibitory ratio and its importance in cell activation. 22 1.5.3. Structure of the FcγR. 23 1.5.4. The FcγR:Fc interaction (binding of IgG to FcγR). 25 1.5.5. Regulation of FcγR expression. 27 1.6. The activatory FcγR. 28 1.6.1. The function of activatory FcγR. 28 1.6.2. Activatory FcγR signalling. 29 1.7. B cell signalling. 32 1.7.1. BCR-induced cytosolic calcium flux. 33 1.7.2. BCR-induced activation of MAP kinase signalling pathway. 34 1.7.3. BCR-induced activation of AKT. 34 1.7.4. The important of lipid rafts in ITAM receptor signalling. 35 iii 1.8. The inhibitory FcγR. 37 1.8.1. Expression of CD32 38 1.8.1.1. Expression of CD32 isoforms. 38 1.8.2. Inhibition of activatory FcγR signalling. 40 1.8.3. Inhibition of BCR signalling. 41 1.8.3.1. CD32 recruitment to lipid rafts after BCR co-ligation. 42 1.8.3.2. Inhibition of BCR-induced cytosolic calcium flux. 43 1.8.3.3. Decreased survival by inhibition of AKT. 43 1.8.3.4. Reduced cell proliferation by inhibition of MAP kinase pathways. 44 1.8.4. Direct B-cell apoptosis induced by CD32 activation. 46 1.9. Expression of FcγR in cancer. 48 1.10. FcγR as mediators of anti-tumour immunotherapy. 50 1.10.1. The role of FcγR in immunotherapy. 50 1.10.2. Inhibitory FcγR in immunotherapy. 50 1.11. Project aims. 52 CHAPTER 2 Materials and Methods 53 2.1. Animals. 53 2.2. Tissue culture. 53 2.3. Cell quantification. 54 2.4. B cell purification by MACS® separation. 54 2.5. Lymphoprep of whole blood. 55 2.6. Mouse genotyping by polymerase chain reaction (PCR). 55 2.6.1. Myc genotyping. 56 2.6.2. CD32-/- genotyping. 56 2.6.3. γ-chain-/- genotyping. 56 2.6.4. Bim-/- genotyping. 57 2.7. Mouse phenotyping by immunofluroscence. 57 2.8. Eµ-myc mice; tumour generation, processing and characterisation. 58 2.9. Antibodies and antibody fragments. 58 2.9.1. Generation of antibodies. 58 2.9.2. Generation of F(ab’)2 antibody fragments. 59 2.10. Antibody dialysis. 59 2.11. Measurement of surface antigens by immunofluorescence. 59 2.12. In vitro Apoptosis Assay. 60 2.12.1. AnV/PI staining of lymphoma and B cells. 60 2.12.2. AnV/PI staining of BMDMs. 61 2.13. Calcium flux assay. 61 2.14. Western blot analysis. 62 iv 2.15. mAb immunotherapy. 63 2.16. Tumour tracking. 64 2.17. Ex vivo modulation assay. 64 2.18. In vitro Modulation assays. 64 2.19. Alexa-488 quenching Assay. 65 2.19.1. Alexa-488 labelling of mAb. 65 2.19.2. Quenching assay. 65 2.20. Adoptive transfer of CFSE labelled cells. 66 2.20.1. CFSE labelling of target and non-target splenocytes. 66 2.20.2. Adoptive transfer of target and non-target cells. 66 2.21. BMDMs phagocytosis assay. 66 2.22. ELISA to determine half life in vivo of anti-CD32 mAb. 67 2.23. Production of clodronate liposomes. 68 2.24. Polymerase chain reaction for murine CD32 isoforms. 68 2.24.1. RNA extraction. 69 2.24.2. cDNA production. 69 2.24.3. PCR for murine CD32 isoforms. 69 2.25. BIAcore analysis to determine affinity of anti-CD32 mAb. 69 2.26. Production of immune complexes. 70 CHAPTER 3 In vitro characterisation of the Eµ-myc mouse model 73 3.1. Chapter introduction. 73 3.2. Kaplein Meier survival. 75 3.3. Presentation of the primary tumour. 76 3.3.1. Physical tumour presentation. 76 3.3.2. Histological examination of tissues from tumour bearing mice. 80 3.3.2.1. Histological examination of lymphoid tissue from the C57BL/6 and Eµ-myc mice. 80 3.3.2.2. Histological examination of non-lymphoid tissue from C57BL/6 and Eµ-myc mice. 81 3.3.2.3. Histological examination of tissues from the Eµ-myc/CD32-/- mice. 81 3.4. Primary tumour characterisation. 86 3.4.1. Tumour phenotype. 86 3.4.2. Tumour transplantation into WT C57BL/6 mice. 89 3.5. Eμ-myc and Eµ-myc/CD32-/- cell line characterisation 90 3.5.1. Eµ-myc and Eµ-myc/CD32-/- cell line growth 90 3.5.2. Changes in IgM expression as cells adapt to culture. 91 3.5.3. Response of Eµ-myc cell-lines to etoposide or BCR stimulation 92 3.6. Chapter Discussion 97 v CHAPTER 4 In vitro analysis of anti-CD32 mAb on lymphoma cells 103 4.1. Chapter Introduction. 103 4.2. Generation of anti-CD32 mAb. 103 4.2.1. anti-CD32 mAb binding specificity and affinity. 104 4.2.2. Identification of epitope specificity. 108 4.2.3. The anti-CD32 mAb block immune complex binding. 108 4.3. Activation of CD32. 111 4.3.1. Tyrosine phosphorylation of CD32, SHIP-1 and SHP by anti-BCR antibodies. 111 4.3.2. Tyrosine phosphorylation of CD32 by the anti-CD32 mAb. 112 4.3.3. Tyrosine phosphorylation of SHIP-1 following activation of CD32 by the anti- CD32 mAb. 113 4.3.4. Tyrosine phosphorylation of CD32 by the anti-CD32 mAb whole IgG and F(ab’)2 fragments. 115 4.3.5. Co-ligation of CD32 by AT130-2 and AT130-5 is in a cis fashion.
Details
-
File Typepdf
-
Upload Time-
-
Content LanguagesEnglish
-
Upload UserAnonymous/Not logged-in
-
File Pages290 Page
-
File Size-