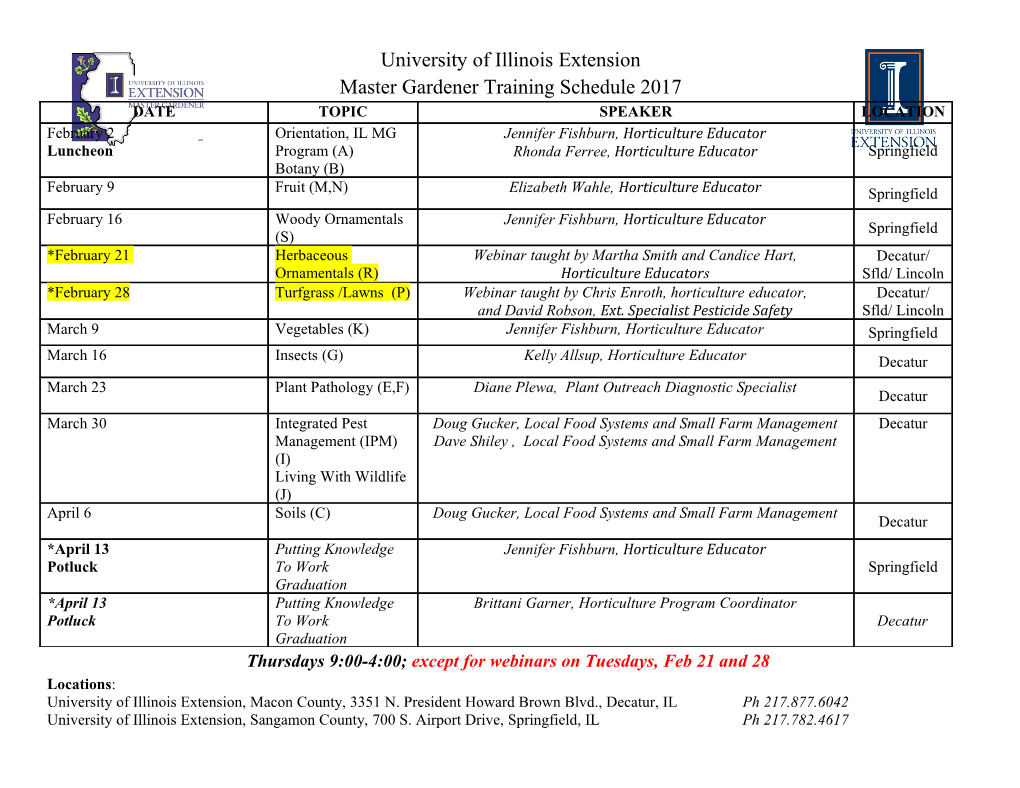
The Astrophysical Journal, 666:231Y235, 2007 September 1 # 2007. The American Astronomical Society. All rights reserved. Printed in U.S.A. STELLAR VELOCITY DISPERSION OF THE LEO A DWARF GALAXY Warren R. Brown, Margaret J. Geller, Scott J. Kenyon, and Michael J. Kurtz Smithsonian Astrophysical Observatory, Cambridge, MA 02138; [email protected], [email protected], [email protected], [email protected] Received 2007 March 28; accepted 2007 May 7 ABSTRACT We measure the first stellar velocity dispersion of the Leo A dwarf galaxy, ¼ 9:3 Æ 1:3kmsÀ1. We derive the velocity dispersion from the radial velocities of 10 young B supergiants and two H ii regions in the central region of ; 7 0 Leo A. We estimate a projected mass of (8 Æ 2:7) 10 M within a radius of 2 and a mass-to-light ratio of at least 20 Æ 6 M /L . These results imply Leo A is at least 80% dark matter by mass. Subject headings:g galaxies: individual (Leo A) 1. INTRODUCTION can contain massive main-sequence stars, blue supergiant stars, and blue loop stars (e.g., Schulte-Ladbeck et al. 2002). We target The Leo A dwarf galaxy was discovered by Zwicky (1942) the 12 blue plume objects with g0 < 21 (squares and triangles). and is one of the most remote galaxies in the Local Group. Leo A Objects with (g0 r0) > 0 in Figure 1 have colors consistent is gas-rich, with an H i velocity dispersion of 3.5Y9kmsÀ1 and À 0 with foreground stars, ranging from F-type stars at the main- with no observed rotation (Allsopp 1978; Lo et al. 1993; Young sequence turnoff (g0 r0) 0:2 to late M dwarfs (g0 r0) > 1. & Lo 1996). Leo A is also extremely metal-poor, with an abun- À 0 À 0 Stars with (g0 À r0) 1:4 may include some asymptotic giant dance of 12 þ log O/H ¼ 7:3Y7.4 measured from H ii regions 0 (Skillman et al. 1989; van Zee et al. 2006). branch stars in Leo A. Figure 2 plots the position of every star in Figure 1. For ref- Photometric studies of Leo A reveal both a red and blue plume erence, the ellipses follow Leo A’s observed H i profile, with of stars in its color-magnitude diagram, indicating recent star forma- center 9h59m23:92s, +3044047:6900 (J2000.00), semiminor-to- tion (Demers et al. 1984; Sandage 1986; Tolstoy 1996). Hubble semimajor axis ratio 0.6, and position angle 104 (Young & Lo Space Telescope observations have resolved the stellar popula- 1996). The solid ellipse marks Leo A’s Holmberg radius, a 3:500 tion of Leo A, which shows evidence for numerous epochs of star ¼ (Mateo 1998), and the dotted ellipse with a 8:00 marks the ex- formation spanning billions of years (Tolstoy et al. 1998; Schulte- ¼ tent of Leo A’s H i gas (Young & Lo 1996) and stellar halo Ladbeck et al. 2002; Cole et al. 2007), as well as an old stellar halo (Vansevicˇius et al. 2004). All 12 blue plume candidates are lo- (Vansevicˇius et al. 2004). RR Lyrae variables confirm the pres- cated within 20 of the center of Leo A; probable foreground ence of an 11 Gyr old population and place Leo A at a distance objects are distributed more uniformly across the field. of 800 Æ 40 kpc (Dolphin et al. 2002). Recently, Brown et al. (2006) reported the first spectroscopy of stars in Leo A, two B 2.2. Observations supergiants stars observed serendipitously as part of their hyper- We obtained spectroscopy of the 12 blue plume objects with velocity star survey. The B supergiants provide spectroscopic proof the 6.5 m MMT telescope and the Blue Channel spectrograph. of star formation as recently as 30 Myr ago in Leo A. Observations occurred during the course of our hypervelocity star Inspired by the B supergiant observations, we have obtained survey program on the nights of 2005 December 5Y6, 2006 spectroscopy for 10 additional blue plume objects in Leo A. There May 24Y25, 2006 June 20, 2006 December 27, and 2007 March 18. isnoapriorireasontoexpectthatLeoA’sstellarandHi gas ve- We operated the Blue Channel spectrograph with the 832 line mmÀ1 locity dispersions are identical. Detailed H i maps show velocity grating in second order and with a 1.2500 slit. These settings pro- structure, which suggests that the gas may be affected by cooling vided a wavelength coverage of 3650Y4500 8 and a spectral reso- or may not yet be relaxed (Young & Lo 1996). Our observations lution of 1.2 8. One object (an H ii region) was reobserved with the allow us to measure the stellar velocity dispersion and thus esti- 300 line mmÀ1 grating and a 1 00 slit, providing wavelength cov- mate the mass of Leo A’s dark matter halo. In x 2wediscussour erage from 3400 to 8600 8 with a spectral resolution of 6.2 8. target selection, observations, and stellar radial velocity determi- Exposure times were 30 minutes. We obtained comparison lamp nations. In x 3 we present the resulting velocity dispersion and exposures after every exposure. The wavelength solutions are mass-to-light ratio of Leo A. We conclude in x 4. determined from 44 lines with typical root mean square residuals 2. DATA of Æ0.05 8,orÆ4kmsÀ1. We note that the single-slit spectro- graph is a compact instrument with minimal flexure: wavelength 2.1. Target Selection solutions shift by less than 1 pixel (0.355 8) during a night, eas- We use Sloan Digital Sky Survey (SDSS; Adelman-McCarthy ily measured from individual comparison lamp exposures. et al. 2007) photometry to select candidate Leo A blue plume 2.3. Spectroscopic Identifications stars by color. We illustrate our target selection in Figure 1, a color-color diagram of every star in SDSS Data Release 5 with Ten blue plume objects are stars of B spectral type and two are g0 < 21 and within 90 of Leo A (see also Fig. 2). We compute de- H ii regions. Figure 3 plots the spectra of the 10 stars and the two reddened colors using extinction values obtained from Schlegel H ii regions, summed and shifted to the rest frame. The signal-to- et al. (1998); the adopted extinction values are E(u0 À g0) ¼ noise ratios (S/N) of the individual spectra range from S/N ¼ 6 0 0 0 0 8 0:029 and E(g À r ) ¼ 0:022. Objects with (g À r )0 < 0and to 15 per pixel at 4000 and depend on the target’s apparent mag- 0 0 (u À g )0 < 1:1 are objects in the blue plume. The blue plume nitude and the seeing conditions of the observation. 231 232 BROWN ET AL. Vol. 666 Fig. 1.—Color-color diagram of every star in SDSS with g0 < 21 and within Fig. 3.—MMT spectra of the 10 B supergiants (top) and the two H ii regions 90 of Leo A (centered at 9h59m23:92s; þ3044047:6900 [J2000.0]). We target the (bottom), summed together and shifted to the rest frame. The continuum fluxes 0 0 12 blue plume candidates with (g À r )0 < 0. We identify 10 B supergiants are arbitrarily normalized. (squares) and two H ii regions (triangles). the 10 stars are likely B supergiants in Leo A. Such B supergiants The 10 B-type stars have visibly narrower Balmer lines and have ages ranging from 30 Myr for the most luminous stars to thus lower surface gravity than the other B-type stars in the Brown 200 Myr for the least luminous stars (Schaller et al. 1992). et al. (2006, 2007) hypervelocity star survey. Cross-correlation with MK spectral standards (Gray et al. 2003) indicates that the 2.4. Radial Velocities stars are probably luminosity class I or II B supergiants, consistent We measure radial velocities with the cross-correlation pack- with the stars’ inferred luminosities. age RVSAO (Kurtz & Mink 1998). We begin by observing the At the distance modulus of Leo A (m M ) 24:51 0:12 À 0 ¼ Æ B9 II star Lyr by quickly scanning the star across the spectro- (Dolphin et al. 2002), the 10 B-type stars have absolute magni- graph slit. This procedure provides us with a very high signal-to- : tudes ranging from MV ¼5 3toÀ3.4. For comparison, Corbally noise ratio cross-correlation template with a known velocity (Evans & Garrison (1984, p. 277) give absolute magnitudes M 5:5 V ¼ 1967; Gray et al. 2003). The accuracy of the velocity zero point : for a B9 Ib star and MV ¼3 1 for a B9 II star. We conclude that comes from the error on the mean of the 44 comparison lamp lines used to determine the template’s wavelength solution, Æ0.6 km sÀ1. It is important that we maximize velocity precision for our velocity dispersion measurement, and we achieve the best preci- sion by cross-correlating the stars with themselves. Thus, after measuring the stars’ velocities with the Lyr template, we shift the spectra to the rest frame and sum them together to create a second template (shown in Fig. 3). We then cross-correlate the 10 stars with this second template of themselves. Table 1 lists the resulting heliocentric radial velocities and errors. The mean cross-correlation precision is Æ3.7 km sÀ1. We also measure the radial velocities of the H ii regions with RVSAO, but this time using Gaussian fits to the emission lines. The final velocity of SDSS J095927.532+304457.75 comes from a weighted mean of the 3727 [O ii] doublet (resolved in our spectra), H,andH emission lines.
Details
-
File Typepdf
-
Upload Time-
-
Content LanguagesEnglish
-
Upload UserAnonymous/Not logged-in
-
File Pages5 Page
-
File Size-