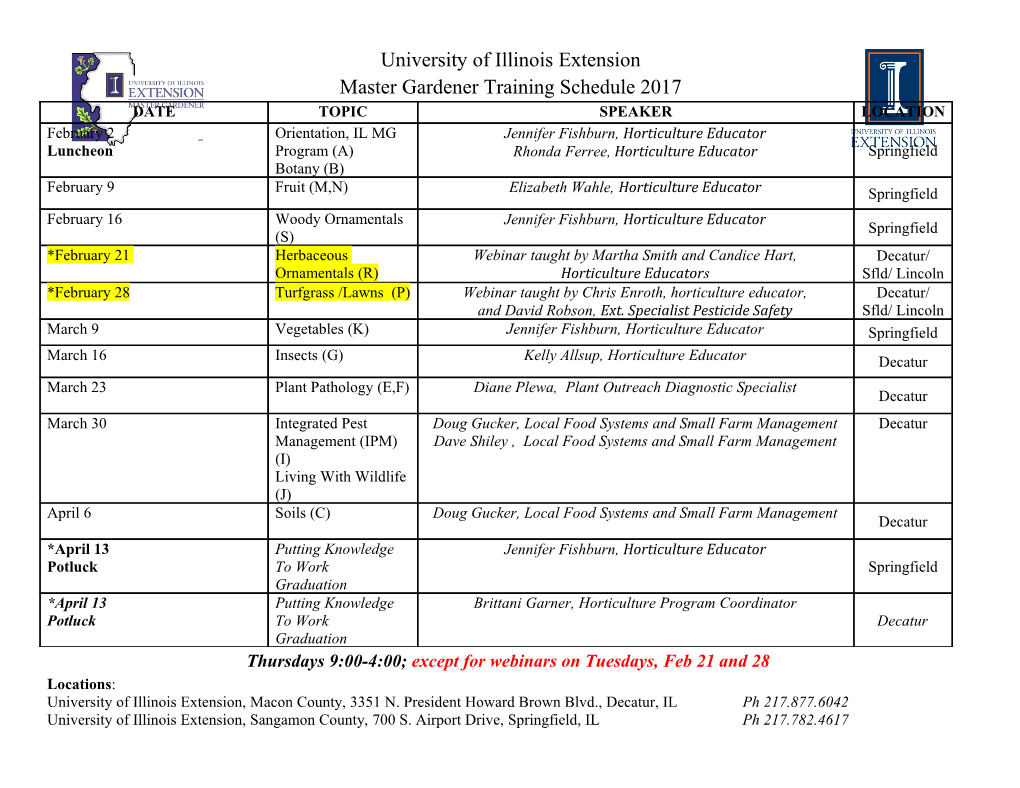
Biochem. J. (1994) 303, 1-14 (Printed in Great Britain) 1 REVIEW ARTICLE Transcriptional control of genes that regulate glycolysis and gluconeogenesis in adult liver Frederic P. LEMAIGRE and Guy G. ROUSSEAU* Hormone and Metabolic Research Unit, University of Louvain Medical School and International Institute of Cellular and Molecular Pathology, 75 Avenue Hippocrate, B-1200 Brussels, Belgium INTRODUCTION listed in Table 1. The ubiquitous factors that control the genes discussed here are listed in Table 2. These genes code for Glycolysis is the metabolic pathway through which glucose is glucokinase, 6-phosphofructo-2-kinase (PFK-2)/fructose-2,6- converted into pyruvate, with a net yield of 2 mol of ATP and bisphosphatase (FBPase-2), pyruvate kinase and phosphoenol- NADH per mol of glucose used. In liver, where the glycolytic pyruvate carboxykinase (PEPCK), namely enzymes that control flux is low except when glucose concentrations are high or key steps, and for aldolase and glyceraldehyde-3-phosphate during anoxia, the main function of glycolysis is to provide dehydrogenase (GAPDH), which are enzymes controlling re- substrates for anabolic processes. While glycolysis occurs in versible steps. every tissue, gluconeogenesis is specific for the liver, the kidney In the present paper, we will review the data pertaining to the and the small intestine. Gluconeogenesis supplies glucose to the transcriptional control of these genes in liver. We will describe body and provides a way to dispose of amino acids and lactate first the glycolytic enzymes and then the gluconeogenic enzyme produced by erythrocytes and during muscle contraction. It is PEPCK. Aldolase B and GAPDH are discussed with the also a means of disposal for glycerol produced during lipolysis. glycolytic enzymes, as they are hormonally controlled like the Liver glycolysis and gluconeogenesis share several enzymes latter. The genes that code for the other enzymes of glycolysis which catalyse reactions that are close to equilibrium (reversible) and gluconeogenesis have not been studied in sufficient detail at under physiological conditions. On the other hand, three sub- the transcriptional level to warrant consideration here. We will strate cycles, i.e. the glucose/glucose 6-phosphate, fructose focus on the relevant cis-acting regions and trans-acting factors 6-phosphate/fructose 1,6-bisphosphate and phosphoenol- in an attempt to assign them a role in the liver-specific tran- pyruvate/pyruvate cycles, involve exergonic reactions that regu- scription and (or) in the response to hormones and carbohydrates. late the pace of glycolysis and gluconeogenesis. These reactions, which are maintained far from equilibrium, are catalysed by GLUCOKINASE different enzymes in the glycolytic and gluconeogenic pathways. Hexokinases (EC 2.7.1.1.) catalyse the phosphorylation of glu- not these key enzymes are the main It is therefore surprising that cose to glucose 6-phosphate. The liver contains four hexokinases Short-term regulation involves targets of regulatory mechanisms. (A to D or I to IV), glucokinase (hexokinase D or IV) being by or and the both the supply of glycolytic gluconeogenic substrates far the predominant isoenzyme. Pancreatic cells also contain a control of the catalytic properties of the enzymes through glucokinase activity. However, the regulation of hepatic and f- Long-term regu- allosteric changes and phosphorylation [1,2]. cell glucokinases differs in a way related to the functions of the lation in liver involves changes in gene expression and protein two cell types. Whereas hepatic glucokinase modulates glucose present review. synthesis [3,4] and is the subject of the uptake, fl-cell glucokinase may play the role of a glucose sensor Indeed, several mechanisms that restrict gene transcription to (reviewed in [5]). By controlling the rate of the glycolytic flux in the liver and modulate it upon hormonal stimulation and nutrient f, cells, glucokinase determines the ATP:ADP ratio, which is supply have been unravelled. These mechanisms rely upon the postulated to affect insulin secretion. Hepatic and f-cell gluco- factors, with interaction of nuclear proteins, called trans-acting kinases therefore play a complementary role in glucose homoeo- cis-acting DNA sequences that belong to the gene considered. stasis. In liver, the regulatory protein of glucokinase binds to and These sequences usually lie upstream from the transcription inhibits glucokinase in the presence of fructose 6-phosphate initiation (cap) site, in the promoter proper or at a distance that (reviewed in [6]). may reach several kilobases, in which case they are called enhancers (or silencers). The latter are sometimes found down- Gene organization stream from the cap site. Trans-acting factors are ubiquitous or 'tissue-enriched', and are distinct from the general transcription The differential regulation of liver and ,-cell glucokinases relies factors that directly assist RNA polymerase II through binding on a genic basis (reviewed in [7]). The liver and ,-cell mRNAs are in the vicinity of the cap site. Some of them are hormone identical with the exception of their 5' end that codes for a receptors, the activity of which is triggered by ligand binding. different 15-amino-acid stretch. In the rat [8,9] and in the human The five, well-characterized, classes of liver-enriched factors are [10,11], there is a single glucokinase gene containing 11 exons Abbreviations used: AF, accessory factor; b-HLH, basic region/helix-loop-helix; C/EBP, CCAAT/enhancer-binding protein; COUP-TF, chicken ovalbumin upstream promoter-transcription factor; CRE, cyclic AMP response element; CREB, cyclic AMP response element-binding protein; DBP, D- binding protein; FBPase-1, fructose-1,6-bisphosphatase; FBPase-2, fructose-2,6-bisphosphatase; GAPDH, glyceraldehyde-3-phosphate dehydro- genase; GIRE, glucose/insulin response element; GRU, glucocorticoid-responsive unit; HNF, hepatocyte nuclear factor; IRE, insulin response element; IRS, insulin response sequence; NF-I, nuclear factor-I; NFY, nuclear factor Y; Oct-1, octamer factor-1; PEPCK, phosphoenolpyruvate carboxykinase; PFK-1, 6-phosphofructo-i-kinase; PFK-2, 6-phosphofructo-2-kinase; PKA, cyclic AMP-dependent protein kinase; PKC, protein kinase C; RAR, retinoic acid receptor; RARE, retinoic acid response element; STAT, signal transducer and activator of transcription; T3, tri-iodothyronine; T3R, T3 receptor; USF, upstream stimulating factor. * To whom correspondence should be addressed. 2 F. P. Lemaigre and G. G. Rousseau C o~~~~~~~0o c- C_ C C) Cu _< o oo E E -. C -C - O >1 0M. co m m m ._! -M -o o ,o CD C. 5 CCCD.C. _nC3O D Q Q x c CD C 2 .= .= .= 2 : C)) CUO CD, C) C C.um=)) )- ) ) 'C) C)) C/) a CD0 QL- a, a, CD CD E C I Co U- LL ^E E LL. Z LL O uj U C? E CY)J Em C-n §= o= L) w CD OC° - (0 O _ LUL5 C) LL. C\i -7L LLC LC 8 14 w -M C .O E LL.LLL. u~~~~~~LLL C LU- c-r.LU LLJ CLi 3 C) C=3 C) C) CD CD .2 ct C) Cssa) C)c Cu C C 11 co 5CD C) UJ C) C) C C)a 0n 0 0n 0n cn 0 CU CU CU CU CU C C S Fa Fo F Fa FZ F ,002 ,0C.2 0= C_ 2 >1 >. 75 = _ cm g C> C cm1 Ca 2Co CO CO C C2 C) 0 0 0 0 C- C~0.0I.C- C. C- C/) U- c, LV Fo F C) Ca U- E ._0 ° ': a) ': °CD o E a.>E a0, E- E Co 0 = o . D 0 a CD _z 6 EI~E E cs E X: ImIUE s E o r, ._ 2 cn~ CIO ~ L Ca = E C) 2 ° ° C.).o2', cm C) > E .h a) E~3 ED 6 , m ._.Ca E c c E EE E C) EC:) C= CDU EEC .- O °Q. O 2 O 0-ILL= Orr1 =I CD., '4 0 co C, CD CD b- CD3 <: @3 cn co C,) CD *0 CD ,.c CD, @3 Z CD n <: < cm cn .-. C3 - C C Ca oD < co cn CD < C) Z CD @3- CD -2- CD CD O CD CD C) Z -: -:t @3 *C~~~~~~~~~~~- a CD = a9 CD CD .4 CD ,_ cn V) ar Is CD CD F- CD7 C= C)~~~~~~~~~~C t) ECD- cm ~~~~~~~~~~a, cn C$C.' t5 ~~~~~~~~~~C) 0 Eo~~~~~~~E°EcmoC) CD _L 3- C) -3 E 'CIO X) Ca ° C=7 IT 0-m C E aC Cu I- LL LLi LL cc CIO Zm Z- C, C/ C, < g.Z LL. I C, I OD I Control of genes that regulate glycolysis and gluconeogenesis 3 and two promoters. The upstream promoter is functional in fi acts co-operatively on the glucokinase gene with the mediator of cells and in the pituitary [8,12], while the downstream promoter insulin action. A similar mechanism regulates the glucocorticoid- functions only in liver [9]. Tissue-specific promoter activity inducibility of the al-acid glycoprotein gene [24]. The effect of produces a liver-type primary transcript that is spliced to yield an insulin might also involve protein kinase C (PKC), since a PKC mRNA containing exons lh and 2 to 10, and a f-cell primary inhibitor blocks insulin stimulation [21]. However, phorbol esters transcript that is spliced to yield an mRNA containing exons 1, do not mimic the effects of insulin [7]. In cultured hepatocytes, and 2 to 10. The organization of the gene therefore explains the dexamethasone and tri-iodothyronine (T3) enhance the stimu- tissue-specific structure of the glucokinase mRNAs. Several lation by insulin [21,25]. minor forms are generated by alternative splicing (reviewed in fl-Cell glucokinase is not sensitive to insulin and its mRNA [7,13]) and some of them code for proteins devoid of glucose levels are not affected by variations in extracellular glucose phosphorylating activity.
Details
-
File Typepdf
-
Upload Time-
-
Content LanguagesEnglish
-
Upload UserAnonymous/Not logged-in
-
File Pages14 Page
-
File Size-