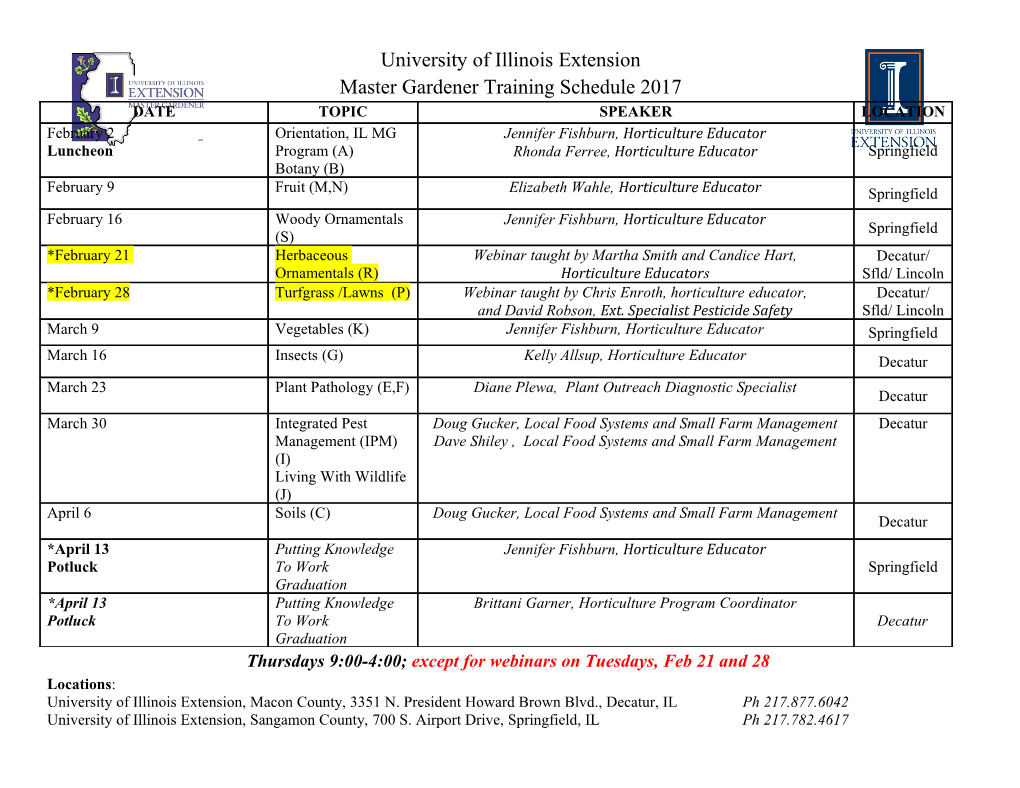
Oncogene (2003) 22, 5848–5854 & 2003 Nature Publishing Group All rights reserved 0950-9232/03 $25.00 www.nature.com/onc Radiation-induced genomic instability and its implications for radiation carcinogenesis Lei Huang*,1,2, Andrew R Snyder2,3 and William F Morgan2,4 1Graduate Program in Human Genetics, University of Maryland, 655 W. Baltimore St., Baltimore, MD 21201-1559, USA; 2Radiation Oncology Research Laboratory, Bressler Research Building, Rm 7-002, University of Maryland, 655 W. Baltimore St., Baltimore, MD 21201-1559, USA; 3Molecular and Cell Biology Graduate Program, University of Maryland, 655 W. Baltimore St., Baltimore, MD 21201-1559, USA; 4Greenebaum Cancer Center, University of Maryland, 655 W. Baltimore St., Baltimore, MD 21201-1559, USA Radiation-induced genomic instability is characterized by lated demonstrating that those same deleterious effects an increased rate of genetic alterations including cytoge- can occur in the progeny of irradiated cells at delayed netic rearrangements, mutations, gene amplifications, times after radiation exposure. These deleterious effects transformation and cell death in the progeny of irradiated occurring in the progeny of an irradiated cell are cells multiple generations after the initial insult. Chromo- broadly grouped under the all-embracing rubric of somal rearrangements are the best-characterized end radiation-induced genomic instability and have been point of radiation-induced genomic instability, and many the subject of a number of recent reviews (Morgan et al., of the rearrangements described are similar to those found 1996; Wright, 1998; Little, 2000). An example of a single in human cancers. Chromosome breakage syndromes are cell clonally expanded after radiation exposure that defined by chromosome instability, and individuals with exhibits genomic instability as determined cytogeneti- these diseases are cancer prone. Consequently, chromo- cally is presented in Figure 1. During clonal expansion somal instability as a phenotype may underlie some of that single irradiated cell, the chromosomes recom- fraction of those changes leading to cancer. Here we bine to generate multiple subpopulations of cells attempt to relate current knowledge regarding radiation- showing unique cytogenetic rearrangements within the induced chromosome instability with the emerging mole- expanded population. cular information on the chromosome breakage syn- dromes. The goal is to understand how genetic and epigenetic factors might influence the onset of chromo- some instability and the role of chromosomal instability in Radiation-induced genomic instability carcinogenesis. Oncogene (2003) 22, 5848–5854. doi:10.1038/sj.onc. 1206697 The current in vogue hypothesis to explain radiation- induced genomic instability is that radiation can initiate Keywords: ionizing radiation; chromosome instability; a process in a cell that can be communicated to other chromosome breakage syndromes; epigenetic factors cells and cause a cascade of cellular events that results in the destabilized genome (Watson et al., 1997, 2000; Lorimore et al., 1998, 2001; Morgan et al., 2002). This can be perpetuated over time by a number of processes involving reactive oxygen species (Clutton et al., 1996; Limoli et al., 1998, 2001; Karanjawala et al., 2002), cell- Introduction to-cell gap junction communication (Azzam et al., 1998, 2001), dead and dying cells in the unstable population The biological effects of ionizing radiation in mamma- (Limoli et al., 1998) and/or secreted factors from lian cells include gene mutation, chromosomal rearran- unstable cells (Nagar et al., 2003). Radiation-induced gement, cellular transformation, cell death and genomic instability appears to be independent of the P53 carcinogenesis. For many years, the central dogma in status of the irradiated cell (Kadhim et al., 1996), but a radiobiology has been that the nucleus, specifically the number of genetic factors influence expression of the DNA, is the principal target for the biological effects of unstable phenotype (Paquette and Little, 1994; Pon- radiation. Following irradiation, the initial radiation- naiya et al., 1997; Watson et al., 1997). Particularly induced DNA damage is converted into a mutation or important among these genetic factors is the target cells’ chromosomal aberration during subsequent DNA re- ability to respond to the initial radiation insult, as pair and is expressed by the irradiated cell and its studies suggest that the DNA repair genes XRCC2 progeny. Over the last 10 years, evidence has accumu- and XRCC3 genes (Cui et al., 1999) and the catalytic subunit of DNA PK (Okayasu et al., 2000; Yu et al., *Correspondence: L Huang; E-mail: [email protected] 2001) may play important roles in maintaining Radiation-induced genomic instability L Huang et al 5849 a cytogenetically visible changes such as chromosome losses or gains, translocations and deletions. It is well known that specific translocations in leukemias and solid tumors lead to the activation of proto-oncogene products or, more commonly, creation of tumor-specific fusion proteins. Multiple chromosomal abnormalities have been described in a variety of human cancers (Mitelman et al., 1997; Mitelman, 2000). The pattern of Radiation exposure clonal expansion abnormalities varies greatly between malignancies, ranging from simple balanced rearrangements to com- plex abnormalities affecting both chromosome structure b and number. Cells with such alterations may display deregulation of the cell cycle and a high mutation rate, which account for the mutator phenotype observed in many cancers and can provide selective growth advan- tages to some tumor cells (Cahill et al., 1999). Thus, abc d genomic instability is a dynamic process that continu- ously modifies the genome of tumor cells over time. The importance of chromosomal instability in tumor progression and its association with cancer highlights the importance of understanding the mechanisms by e f g which they arise. The mechanisms are likely complex and involve numerous cellular processes including Figure 1 Schematic of radiation-induced genomic instability. (a) mitotic checkpoint regulation (Cahill et al., 1998), Cells are irradiated, and a surviving cell is clonally expanded. DNA-damage signaling and repair (Khanna and Jack- During clonal expansion, radiation-induced genomic instability results in chromosome rearrangements occurring in the progeny of son, 2001), as well as telomere maintenance and the irradiated cells, giving rise to heterogeneous subpopulations of centrosome function (Kramer et al., 2002; Maser and cytogenetically different cells, represented by the different colored DePinho, 2002). Recently, a number of exciting research circles, within the clone. Instability can also lead to aneuploidy, advances have suggested a role for nontargeted epige- delayed reproductive cell death, giant cell formation, gene amplification and/or increased mutations in these same cell clones netic effects in perpetuating radiation-induced genomic (Limoli et al., 1997a). (b) Chromosomal instability in a human- instability. In this review, we will compare radiation- hamster hybrid GM10115 clone. GM10115 cells contain a single induced chromosomal instability with the chromosome copy of human chromosome 4, which is identified by fluorescence fragility syndromes in order to explore potential under- in situ hybridization and serves as a maker for induced instability lying mechanisms that drive the dynamic production (Marder and Morgan, 1993). Metaphase chromosomes are ‘painted’ with a fluorescence-labeled probe for the telomere of novel chromosomal rearrangements that charac- (TTAGGG, red) and a human chromosome 4-specific DNA terize chromosomal instability and contribute to carci- library (green). The hamster chromosomes are counterstained with nogenesis. DAPI (blue). (a) A normal, nonrearranged metaphase cell with B14 interstitial telomere repeat-like sequences hybridizing red on the hamster chromosomes and the human chromosome fluorescing Chromosome breakage syndromes green. (b–g) Different subpopulations of metaphase chromosomes from an unstable clone showing unique rearrangements of the The chromosome breakage syndromes, ataxia–telan- human chromosome with the hamster genome some of which, for giectasia, Nijmegen breakage syndrome, ataxia–telan- example (b, d, e), involve recombination junctions involving the giectasia-like disorder, Bloom syndrome, Werner interstitial telomere repeat-like sequences at the junction between the human and hamster genomes (Day et al., 1998) syndrome and Fanconi anemia are human autosomal recessive diseases characterized by inherited chromoso- mal instability and cancer predisposition. (Taylor, 2001; Duker, 2002; Thompson and Schild, 2002). The path- chromosome stability after cellular exposure to ionizing ways linking the genetic defect to the eventual develop- radiation. ment of various neoplasms remain unknown, but the chromosomal instabilities and neoplastic outcomes are Genomic instability in cancer related to specific genetic defects in DNA replication, DNA repair, cell-cycle checkpoint, or control of The loss of stability of the genome is now widely apoptosis. Ataxia–telangiectasia, Nijmegen breakage accepted as one of the most important aspects of cancer syndrome and ataxia–telangiectasia-like disorder are (Lengauer et al., 1998; Coleman and Tsongalis, 1999). defective in the ataxia–telangiectasia mutated (ATM) Nowell (1976) suggested that genomic instability within (Lavin and Shiloh, 1997), NBS1
Details
-
File Typepdf
-
Upload Time-
-
Content LanguagesEnglish
-
Upload UserAnonymous/Not logged-in
-
File Pages7 Page
-
File Size-