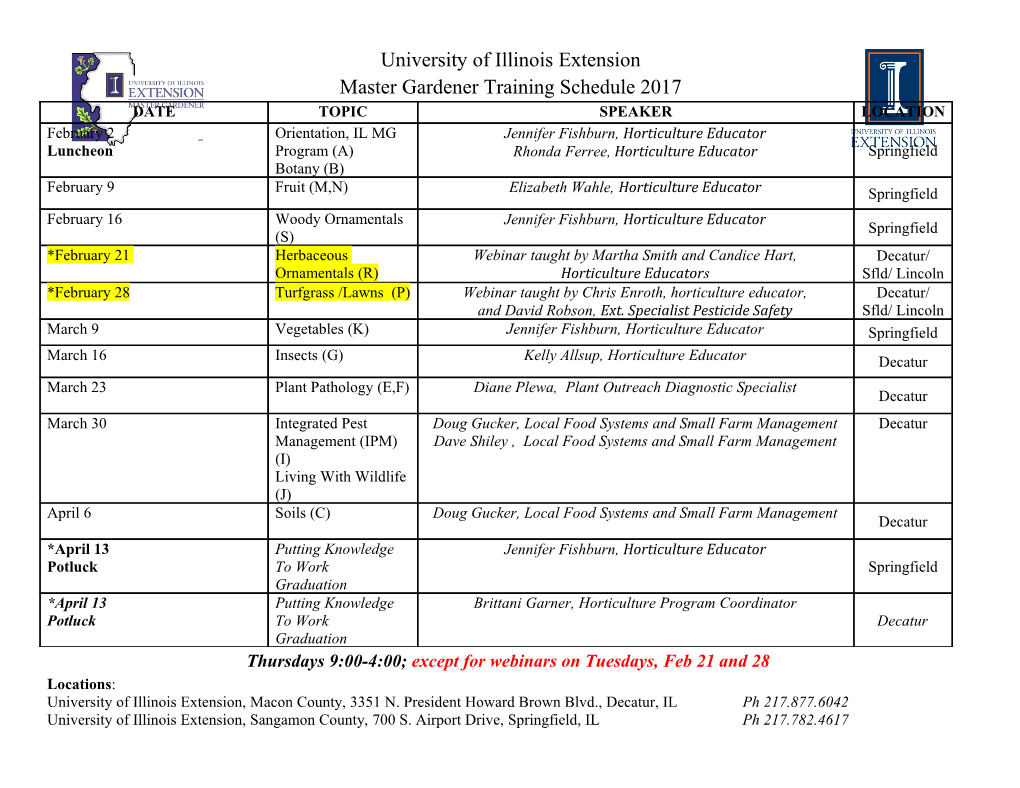
University of Rhode Island DigitalCommons@URI Biological Sciences Faculty Publications Biological Sciences 2015 The ffecE t of Light Intensity on Prey Detection Behavior in Two Lake Malawi Cichlids, Aulonocara stuartgranti and Tramitichromis sp. Margot A.B. Schwalbe Jacqueline F. Webb University of Rhode Island, [email protected] Follow this and additional works at: https://digitalcommons.uri.edu/bio_facpubs The University of Rhode Island Faculty have made this article openly available. Please let us know how Open Access to this research benefits oy u. This is a pre-publication author manuscript of the final, published article. Terms of Use This article is made available under the terms and conditions applicable towards Open Access Policy Articles, as set forth in our Terms of Use. Citation/Publisher Attribution Schwalbe MA, Webb JF. (2015). "The Effect of Light Intensity on Prey Detection Behavior in Two Lake Malawi Cichlids, Aulonocara stuartgranti and Tramitichromis sp." Journal of Comparative Physiology A: Neuroethology, Sensory, Neural, and Behavioral Physiology. Available at: http://link.springer.com/article/10.1007/s00359-015-0982-y# This Article is brought to you for free and open access by the Biological Sciences at DigitalCommons@URI. It has been accepted for inclusion in Biological Sciences Faculty Publications by an authorized administrator of DigitalCommons@URI. For more information, please contact [email protected]. 1 2 The effect of light intensity on prey detection behavior in two Lake Malawi cichlids, 3 Aulonocara stuartgranti and Tramitichromis sp. 4 5 6 7 8 Margot A. B. Schwalbe1 and Jacqueline F. Webb1 9 10 1Department of Biological Sciences, University of Rhode Island, 120 Flagg Road, Kingston, RI 11 02881, USA 12 13 14 Author for Correspondence: Margot A. B. Schwalbe, Department of Biology, Tufts University, 15 200 Boston Ave, Ste. 4700, Medford, MA USA; [email protected], 16 [email protected], telephone: (617) 627-0558, fax: (617) 627-0309 17 18 19 Tables: 5 20 Figures: 7 (2 color) 21 22 23 1 24 Abstract 25 26 Two Lake Malawi sand-dwelling cichlids (Aulonocara stuartgranti, Tramitichromis sp.) that 27 have different lateral line phenotypes, but feed on benthic invertebrates, have been shown to use 28 lateral line and/or visual cues to detect prey under light versus dark conditions. The current study 29 examined how ecologically relevant variation in light intensity (0-800 lux) influences detection 30 of prey (mobile, immobile) in each species by analyzing six well-defined behavioral parameters. 31 Both species fed at light intensities ≥1 lux; prey type and/or time of day (but not light intensity) 32 predicted all four parameters analyzed with generalized linear mixed models in A. stuartgranti, 33 whereas the interaction of light intensity and time of day predicted three of these parameters in 34 Tramitichromis sp. Data for all six parameters suggest that the critical light intensity is 1-12 lux 35 for both species, the integration of visual and lateral line input explains differences in the 36 detection of mobile and immobile prey and the behavioral changes that occur at the transition 37 from 1 to 0 lux in A. stuartgranti, and that Tramitichromis sp. likely uses binocular vision to 38 locate prey. The sensory biology of species that exploit similar food resources will have 39 important implications for the trophic ecology of African cichlid fishes. 40 41 Keywords 42 Vision, lateral line, detection distance, prey detection, sensory ecology 43 44 Abbreviations 45 AICC Akaike information criterion 46 GLMM Generalized linear mixed model 2 47 Lx Lux 48 PAR Photosynthetically active radiation 49 SL Standard length 50 TL Total length 51 52 Introduction 53 54 Light in aquatic habitats varies in quality and quantity over time and space (Kirk 2011) and 55 influences the ability of visual predators to detect and capture mobile prey (Vinyard and O’Brien 56 1976; Confer et al. 1978; Lythgoe 1979; Ryer and Olla 1999; Vogel and Beauchamp 1999; 57 Rickel and Genin 2005). Fishes occupying similar habitats may demonstrate variation in 58 visually-mediated prey detection abilities, such as visual thresholds and absorption spectra of 59 visual pigments, which may provide a competitive advantage under particular light conditions 60 (Vogel and Beauchamp 1999; Hofmann et al. 2009). Many fishes are also able to detect prey at 61 low light intensities (e.g., dawn, dusk, at depth, or with increased turbidity), but with reduced 62 capabilities compared to that at higher light intensities. The distance at which free swimming 63 prey are detected dramatically decreases below a certain light intensity (“critical light intensity,” 64 Confer et al. 1978) in salmonids (Dunbrack and Dill 1984; Henderson and Northcote 1985) and 65 some freshwater percomorphs (bluegill, Lepomis macrochirus, Vinyard and O’Brien 1976; 66 largemouth bass, Micropterus salmoides, Howick and O’Brien 1983; yellow perch, Perca 67 flavescans, Richmond et al. 2004). 68 Given the importance of multimodal sensory integration in the formulation of behavior, 69 the contributions of the non-visual sensory systems to prey detection (e.g., mechanosensory 3 70 lateral line, auditory, olfactory, gustatory, somatosensory/tactile, and in some cases, the 71 electrosensory system; reviewed in Montgomery et al. 2014) must also be considered. 72 Morphological and/or physiological specializations of non-visual sensory systems, including the 73 olfactory system (Parzefall 1993; Montgomery et al. 1999), gustatory system (Atema 1971) and 74 the lateral line system (Janssen 1997; Schwalbe et al. 2012, reviewed in Webb 2014), have been 75 used to predict how these senses provide alternatives to vision for prey detection in light-limited 76 environments. Futhermore, the integration of different combinations of sensory inputs may 77 explain variation in behavior under different environmental conditions (Partridge and Pitcher 78 1980; Moller 2002; Montgomery et al. 2003; Gardiner and Motta 2012). Several species of fishes 79 have been shown to modulate feeding strategies using a combination of visual and non-visual 80 cues that allow them to feed under a range of light conditions, including darkness (Townsend and 81 Risebrow 1982; Batty et al. 1986; Diehl 1988; Schwalbe et al. 2012). 82 The mechanosensory lateral line system is known to play important roles in prey 83 detection, as well as in predator avoidance, communication, and navigation around obstacles 84 (Webb et al. 2008; Montgomery et al. 2014). The system demonstrates a great deal of variation, 85 which is defined by the morphology of the cranial and trunk lateral line canals and neuromast 86 receptor organs within them, and the distribution of superficial neuromasts on the skin of the 87 head, trunk and tail (reviewed in Webb 2014). Widened lateral line canals, one of five cranial 88 lateral line canal phenotypes found among bony fishes, has evolved convergently in ~12 teleost 89 families (including deep sea taxa) and appears to be an adaptation for enhanced sensitivity to 90 water flows and prey detection (Denton and Gray 1988, 1989; Montgomery and Coombs 1992; 91 discussed in Schwalbe et al. 2012; reviewed in Webb 2014). 4 92 The speciose cichlid fishes of the African Rift Lakes are typically described as visual 93 feeders (Fryer and Iles 1972) and most genera have narrow cranial lateral line canals, but all 94 members of a few genera (e.g., Alticorpus, Aulonocara, Aulonocranus, Trematocara, 95 Trematocranus, Konings 2007) have widened lateral line canals suggesting the capacity for 96 lateral line mediated prey detection (Konings 1990). Two genera of non-mbuna, haplochromine 97 cichlids in Lake Malawi, Aulonocara (widened canals) and Tramitichromis (narrow canals; Fig. 98 1), provide an interesting taxon pair for comparison of prey detection strategies since both feed 99 on benthic invertebrates in the sand, and thus appear to be ecologically similar. Schwalbe et al. 100 (2012) and Schwalbe and Webb (2014) analyzed the behavioral responses of Aulonocara 101 stuartgranti and Tramitichromis sp. to tethered live and dead prey (=adult brine shrimp, Artemia 102 sp.), in experiments carried out under light and dark conditions in which the lateral line system 103 was experimentally inactivated. These studies demonstrated that A. stuartgranti uses a 104 combination of inputs to its visual and lateral line systems to detect prey in the light, but depends 105 on its lateral line system to detect prey in the dark. Furthermore, these studies showed that 106 deactivation of the lateral line system of A. stuartgranti significantly affected prey detection 107 behavior and revealed that other senses (olfaction, gustation, and somatosensory/tactile) were 108 insufficient to initiate prey detection behavior in the dark. In contrast, Tramitichromis sp. did not 109 feed in the dark, and the inactivation of the lateral line system had little effect on prey detection 110 behavior in the presence of light, demonstrating that it is a visual predator. 111 Aulonocara and Tramitichromis species appear to share a food resource (benthic 112 invertebrates in sandy substrates), but occupy different depth ranges (Aulonocara species at 113 depths of 5-120 m and Tramitichromis species at depths of < 15 m; Fryer and Iles 1972; Konings 114 1990, 2007) and use different strategies to detect and capture benthic invertebrate prey in the 5 115 field. Species of Aulonocara swim just above the substrate to sense hydrodynamic flows 116 generated by benthic invertebrates in or on the substrate in the field (Konings 2007). In contrast, 117 species of Tramitichromis typically capture invertebrate prey by plunging into the substrate, 118 filling their mouth with sand, and sifting out prey with their gill rakers in the field (= “sand 119 sifting,” Fryer, 1959). This sand sifting behavior appears to be synonymous with the 120 “winnowing” behaviors observed in some surfperches (Laur and Ebeling 1983) and vision likely 121 contributes to the ability to locate patches of high quality food resources where “winnowing” 122 takes place (Holbrook and Schmitt 1984; Schmitt and Holbrook 1984).
Details
-
File Typepdf
-
Upload Time-
-
Content LanguagesEnglish
-
Upload UserAnonymous/Not logged-in
-
File Pages50 Page
-
File Size-