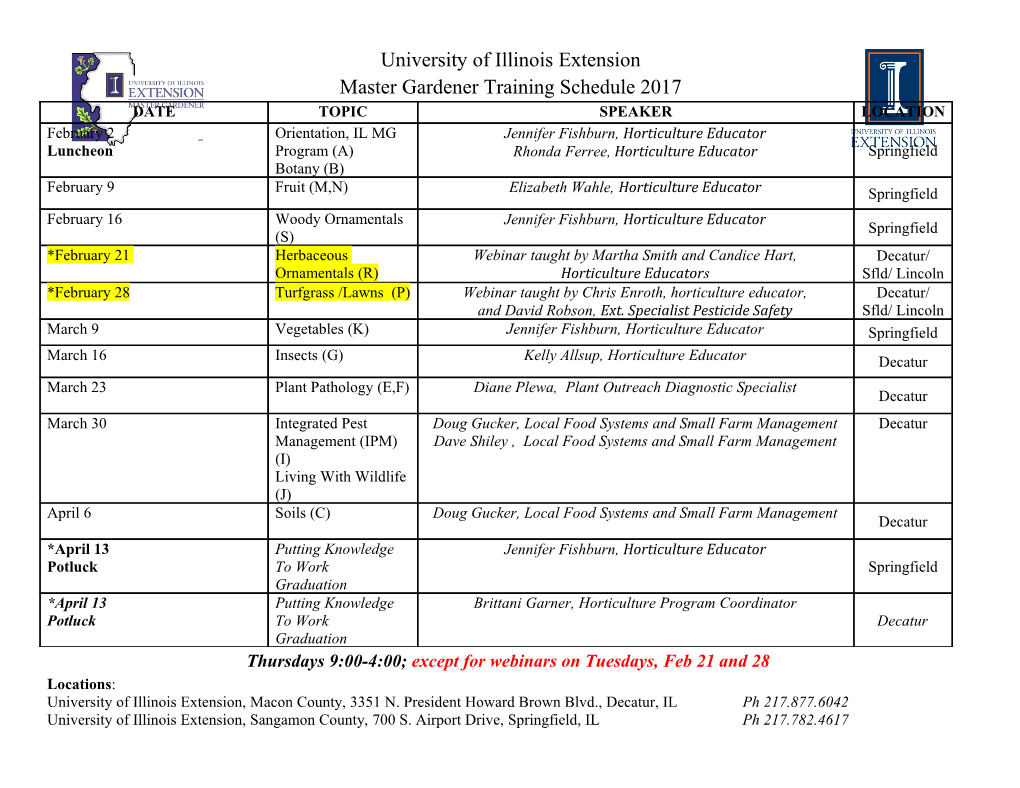
Article Negative feedback control of neuronal activity by microglia https://doi.org/10.1038/s41586-020-2777-8 Ana Badimon1,2,3, Hayley J. Strasburger1,2,3, Pinar Ayata1,2,3,4, Xinhong Chen5, Aditya Nair5, Ako Ikegami6,7, Philip Hwang1,2,3, Andrew T. Chan1,2,3, Steven M. Graves8, Joseph O. Uweru9, Received: 26 November 2019 Carola Ledderose10, Munir Gunes Kutlu11, Michael A. Wheeler12, Anat Kahan5, Accepted: 28 August 2020 Masago Ishikawa1, Ying-Chih Wang13, Yong-Hwee E. Loh1, Jean X. Jiang14, D. James Surmeier15, Simon C. Robson16,17, Wolfgang G. Junger10, Robert Sebra13, Erin S. Calipari11,18,19,20,21, Published online: 30 September 2020 Paul J. Kenny1, Ukpong B. Eyo9, Marco Colonna22, Francisco J. Quintana12,23, Hiroaki Wake6,7, Check for updates Viviana Gradinaru5 & Anne Schaefer1,2,3,4 ✉ Microglia, the brain’s resident macrophages, help to regulate brain function by removing dying neurons, pruning non-functional synapses, and producing ligands that support neuronal survival1. Here we show that microglia are also critical modulators of neuronal activity and associated behavioural responses in mice. Microglia respond to neuronal activation by suppressing neuronal activity, and ablation of microglia amplifes and synchronizes the activity of neurons, leading to seizures. Suppression of neuronal activation by microglia occurs in a highly region-specifc fashion and depends on the ability of microglia to sense and catabolize extracellular ATP, which is released upon neuronal activation by neurons and astrocytes. ATP triggers the recruitment of microglial protrusions and is converted by the microglial ATP/ADP hydrolysing ectoenzyme CD39 into AMP; AMP is then converted into adenosine by CD73, which is expressed on microglia as well as other brain cells. Microglial sensing of ATP, the ensuing microglia-dependent production of adenosine, and the adenosine- mediated suppression of neuronal responses via the adenosine receptor A1R are essential for the regulation of neuronal activity and animal behaviour. Our fndings suggest that this microglia-driven negative feedback mechanism operates similarly to inhibitory neurons and is essential for protecting the brain from excessive activation in health and disease. Human and animal behaviour relies on the coordinated activity of in the striatum in response to the selective activation of CAMKII+ excitatory and inhibitory neurons, which collectively define the out- neurons in the mouse forebrain (Fig. 1b, c) indicates that neuronal put of distinct neuronal circuits and associated behaviours. Although activity is communicated to microglia and may alter microglia–neu- the regulation of neuronal activity in the brain has long been viewed as ron interactions. In particular, the upregulation of genes involved in an exclusive prerogative of neurons, recent findings have suggested chemotaxis (Ccl24, Ccl2, Ccl3) and actin filament polymerization that the brain’s immune cells – the microglia – might be involved in this (Kank2, Twf1) (Fig. 1b, Supplementary Table 1), and the downregu- process2–8. We found that, similar to inhibitory neurons, microglia sense lation of genes that govern baseline motility of microglia, such as neuronal activation and suppress excessive neuronal activity. Microglia the key regulator Kcnk13 (also known as THIK-1)9,10 (Fig. 1b, c, respond to neuronal activation or inhibition with distinct changes Supplementary Table 1), suggest that neuronal activation alters neu- in gene expression (Fig. 1a–c, Extended Data Fig. 1, Supplementary ron–microglia interactions by affecting microglial process extension Tables 1, 2). The overall pattern of changes in microglial gene expression and motility11,12. 1Nash Family Department of Neuroscience, Icahn School of Medicine at Mount Sinai, New York, NY, USA. 2Department of Psychiatry, Icahn School of Medicine at Mount Sinai, New York, NY, USA. 3Center for Glial Biology, Icahn School of Medicine at Mount Sinai, New York, NY, USA. 4Ronald M. Loeb Center for Alzheimer’s Disease, Icahn School of Medicine at Mount Sinai, New York, NY, USA. 5Division of Biology and Biological Engineering, California Institute of Technology, Pasadena, CA, USA. 6Department of Anatomy and Molecular Cell Biology, Nagoya University Graduate School of Medicine, Nagoya, Japan. 7Division of System Neuroscience, Kobe University Graduate School of Medicine, Kobe, Japan. 8Department of Pharmacology, University of Minnesota, Minneapolis, MN, USA. 9Center for Brain Immunology and Glia, Department of Neuroscience, University of Virginia, Charlottesville, VA, USA. 10Department of Surgery, Beth Israel Deaconess Medical Center, Harvard Medical School, Boston, MA, USA. 11Department of Pharmacology, Vanderbilt University, Nashville, TN, USA. 12Ann Romney Center for Neurologic Diseases, Brigham and Women’s Hospital, Harvard Medical School, Boston, MA, USA. 13Department of Genetics and Genomic Sciences, Icahn Institute of Data Science and Genomic Technology, Icahn School of Medicine at Mount Sinai, New York, NY, USA. 14Department of Biochemistry and Structural Biology, University of Texas Health Science Center, San Antonio, TX, USA. 15Department of Physiology, Feinberg School of Medicine, Northwestern University, Chicago, IL, USA. 16Department of Anesthesia, Beth Israel Deaconess Medical Center and Harvard Medical School, Boston, MA, USA. 17Department of Medicine, Beth Israel Deaconess Medical Center and Harvard Medical School, Boston, MA, USA. 18Vanderbilt Brain Institute, Vanderbilt University, Nashville, TN, USA. 19Vanderbilt Center for Addiction Research, Vanderbilt University, Nashville, TN, USA. 20Department of Molecular Physiology and Biophysics, Vanderbilt University, Nashville, TN, USA. 21Department of Psychiatry and Behavioral Sciences, Vanderbilt University, Nashville, TN, USA. 22Department of Pathology and Immunology, Washington University School of Medicine, St. Louis, MO, USA. 23The Broad Institute of MIT and Harvard, Cambridge, MA, USA. ✉e-mail: [email protected] Nature | Vol 586 | 15 October 2020 | 417 Article Microglia –0.45 abCNO 0.27 c 1.0 Ccl3 Pathway enrichment of genes upregulated hM3Dq Ccl2 Neuronal Kdm6b in microglia upon neuronal activation + Rgs1 CaMKII neuron excitation Arhgap29 Ccl24 Cebpa Chemotaxis Kank2 Microglia 0.3 Plk3 Positive regulation of Twf1 Plekhg4 GTPase activity Raf1 Cellular response to Lrp4 -score z Plxnb3 cytokine stimulus Adrb1 Regulation of actin eGFP-labelled Anti-GFP –0.3 Vsig10l Mafk lament polymerization microglial polybeadA s Clec1b Lyz1 polysomes Cd9 01 2345 Kcnk13 –log P TRAP Ifit3 10 RNA sequencing – CNO + CNO hM3Dq d e Kainic acid f Picrotoxin g D1 agonist Padj = 0.0006 Control Microglia-decient 100 P = 0.001 100 100 P = 0.005 Padj = 0.0009 80 80 80 60 60 60 100um 40 40 40 20 20 Mice with stage Mice with stage 20 IV–V seizures (%) IV–V seizures (%) 100 μm 100 μm 1/9 9/10 1/10 14/20 7/33 9/11 11/14 0 Mice seizing at 30 min (%) 0 0 IBA1 DAPI IBA1 DAPI trol Control croglia- Con 3 days Control 3 weeksCSF1Ri CSF1Ri Microglia- Mi decient decient Fig. 1 | Microglia respond to neuronal activation and prevent excessive d, Microglia depletion by the CSF1R inhibitor PLX5622. Representative images neurostimulation. a, Transcriptional changes in microglia induced by of sagittal striatal sections from control (left) or PLX5622-treated mice (right) neuronal activity. CaMKII-tTa; tetO-CHRM3 mice expressing the human M3 show nucleated (DAPI+, blue) IBA1+ (green) microglia. e–g, Percentage of mice muscarinic (hM3Dq) receptor in CaMKII+ neurons were bred to Cx3cr1CreErt2/+(Litt); exhibiting behavioural seizures in response to i.p. injections of kainic acid Eef1a1LSL.eGFPL10a/+ mice. Neurons were activated by clozapine-N-oxide (CNO), (e, 18 mg kg−1, 1 h), picrotoxin (f, 1 mg kg−1, 30 min) and D1 agonist (g, SKF81297, followed by microglia-specific mRNA analysis using translating ribosome 5 mg kg−1, 1 h) (e, n = 9 and 10 mice, Fisher’s exact test; f, n = 10 and 20 mice, affinity purification (TRAP). b, Gene expression changes in striatal microglia Fisher’s exact test; g, n = 33, 11, and 14 mice, P < 0.0001, χ2 test with Bonferroni following CNO-induced activation (z-scored log2RPKM; n = 3 mice) c, Selected adjustment). The experiments shown in d, e and g have been independently gene ontology (GO) annotations (using ENRICHR) for genes that were repeated with same results. RPKM, reads per kilobase of transcript per million upregulated (using DESeq2) in striatal microglia (dotted line, P = 0.05). mapped reads; data shown as mean ± s.e.m. We found that the microglial response to neuronal activation is asso- by interleukin-34 (IL34) and colony-stimulating factor 1 (CSF1), both ciated with reciprocal, microglia-mediated suppression of neuronal of which can activate CSF1R signalling and promote the survival of activity. Brain-wide ablation of microglia in adult mice by pharma- microglia15–17. In the striatum, the expression of Il34 and Csf1 is spa- cological inhibition of the microglia pro-survival receptor CSF1R13 tially separated; Il34 is expressed by D1 and D2 medium spiny neurons (Fig. 1d, Extended Data Fig. 2a) had no major effect on animal behav- (MSNs) in the grey matter, whereas Csf1 is predominantly expressed by iours at baseline13 (Extended Data Fig. 2b–e), but rendered animals oligodendrocytes and astrocytes in white matter regions16,18 (Fig. 2a, b, hyper-responsive to neurostimulants at levels that normally do not Extended Data Fig. 3a, b). Ablation of Il34 specifically in neuronal cause excessive neuronal activation (Fig. 1e–g, Extended
Details
-
File Typepdf
-
Upload Time-
-
Content LanguagesEnglish
-
Upload UserAnonymous/Not logged-in
-
File Pages41 Page
-
File Size-